Mastering Cell Line Development: From Basics to Innovations
Imagine you're a scientist on the brink of a breakthrough, striving to develop a revolutionary therapeutic for a complex disease. This journey starts with a crucial step: cell line development (CLD). CLD involves engineering a cell line, typically mammalian, to produce therapeutic biomolecules or biologics. This essential process allows scientists to customize cell cultures based on specific applications and desired properties of the target product. Developing a robust and stable cell line capable of yielding sufficient material for testing, optimization, and commercial-scale manufacturing is a critical phase in bioprocess development. The strategies and decisions made during the early stages of cell line development are pivotal, often determining the project's overall success or failure. In the competitive fields of healthcare and biotechnology, where the production of monoclonal antibodies and therapeutic proteins is crucial, the importance of stable cell line development cannot be overstated. The precision and foresight applied in these early stages shape the trajectory of innovative treatments, ensuring they meet the rigorous demands of clinical applications and large-scale manufacturing. Dive into the fascinating and transformative process of cell line development. This blog post is your comprehensive guide to everything you need to know about cell line development, revealing how these meticulous efforts are paving the way for groundbreaking therapies and life-saving innovations. Let’s begin.
- Overview of Cell Line Development
- Importance of Cell Line Development in Biotechnology and Pharmaceuticals
- Aim of This Blog
- Definition of Cell Line Development
- What is Cell Line Development?
- Historical Background
- Principles of Cell Line Development
- Basic Concepts
- The Cell Line Development Process
- Comprehensive Overview of the Cell Line Development Process
- Methods of Gene Transfection in Cell Line Development
- Overview of Transfection Methods
- Chemical Transfection
- Physical Transfection
- Viral Transfection
- Stable vs. Transient Cell Lines
- Definitions and Differences
- Stable Cell Lines
- Transient Cell Lines
- Challenges and Solutions in Cell Line Development
- Common Challenges
- Strategies to Overcome Challenges
- Comparison of Cell Line Development Techniques
- Comparative Analysis of Different Techniques
- Traditional Techniques
- Modern Techniques
- Pros and Cons of Different Techniques
- Regulatory Considerations
- Compliance with International Standards
- Ethical Considerations
- FAQs and Common Questions
- Conclusion
- Resources and Further reading
Overview of Cell Line Development
Cell line development is a fundamental process in biotechnology and pharmaceutical industries, involving the creation and optimization of cell lines that can produce specific proteins, antibodies, or other therapeutic molecules. This process is crucial for the production of biopharmaceuticals, vaccines, and various research applications. The development of cell lines involves a series of steps, including the selection of appropriate host cells, genetic manipulation, and extensive screening to ensure stability and high yield of the desired product.
Importance of Cell Line Development in Biotechnology and Pharmaceuticals
In the realm of biotechnology and pharmaceuticals, cell line development is indispensable. It underpins the production of monoclonal antibodies, therapeutic proteins, and other critical biomolecules. Stable cell line development is especially vital for producing consistent and high-quality products, which are essential for clinical applications and large-scale manufacturing. Mammalian cell line development, particularly CHO (Chinese Hamster Ovary) cell line development, is widely used due to its ability to produce human-like glycosylation patterns in proteins, making them suitable for therapeutic use.
Aim of This Blog
The aim of this blog is to provide a comprehensive review of cell line development. We will cover the cell line development process, workflow, and methodologies, with a focus on stable cell line development. Additionally, we will explore the specific aspects of CHO cell line development and discuss the latest advancements in the field. By the end of this blog, readers will have a thorough understanding of what cell line development entails and how it can benefit their research and industrial applications.
The journey from research to large-scale production is fraught with hurdles. From unpredictable genetic stability to labour-intensive clone selection, these challenges can slow progress and increase costs. Discover how to overcome these obstacles and optimise your stable cell line development.
Download your essential guide to scalable high-quality bioproduction
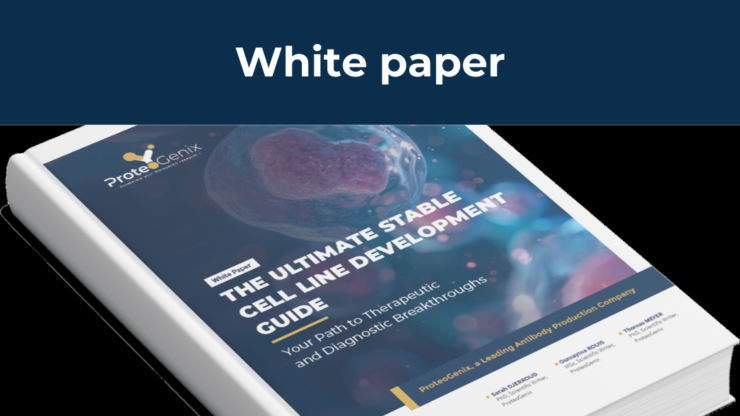
Definition of Cell Line Development
What is Cell Line Development?
Cell line development is the process of creating a stable cell line that can produce a specific therapeutic protein or biomolecule at high levels. This involves the genetic modification of cells, often mammalian cells, to incorporate the gene of interest, followed by rigorous selection and screening to ensure that the cells can consistently and efficiently produce the desired product. The cell line development process is critical for manufacturing monoclonal antibodies, vaccines, and other biopharmaceuticals, ensuring that they are produced with the necessary quality and consistency for therapeutic use.
Modern cell line development typically utilizes Chinese Hamster Ovary (CHO) cells due to their ability to adapt to suspension culture, grow in serum-free media, and produce human-like post-translational modifications. CHO cells are widely regarded for their robustness and suitability for large-scale production of recombinant proteins, making them the preferred choice in the biopharmaceutical industry.
Historical Background
The history of cell line development dates back to the early 20th century, with significant milestones that have shaped the field. One of the earliest breakthroughs was the establishment of the HeLa cell line in 1951 by George Gey. HeLa cells, derived from cervical cancer cells, were the first immortal human cell line and have been instrumental in numerous scientific discoveries.
In the 1980s, the approval of CHO-derived tissue plasminogen activator (tPA) by the US Food and Drug Administration (FDA) marked a pivotal advancement in the use of mammalian cell culture for therapeutic protein production. This approval demonstrated the potential of CHO cells in producing complex proteins with human-like glycosylation patterns, critical for their therapeutic efficacy and safety.
The development of methotrexate (MTX) amplification technology and the glutamine synthetase (GS) system in the 1980s further revolutionized cell line development. These technologies enable the selection of stable cell clones with high productivity by increasing the gene copy number of the target protein within the host cells. Despite the initial challenges of heterogeneity among cell clones, advancements in screening and selection techniques have significantly improved the efficiency and reliability of the cell line development process.
In recent decades, cell line development has continued to evolve with the incorporation of advanced genetic engineering techniques, high-throughput screening methods, and automation. These advancements have reduced the time and cost associated with developing stable, high-producing cell lines, making it possible to meet the increasing demand for biopharmaceutical products.
Principles of Cell Line Development
Basic Concepts
Cell line development involves the creation of cell lines capable of stable and consistent production of specific proteins or biomolecules. This process is underpinned by several fundamental concepts:
- Genetic Engineering: The initial step in cell line development is the integration of the gene of interest into the host cell’s genome. This is typically achieved through transfection methods, which include chemical transfection (using calcium phosphate or liposomes), physical transfection (such as electroporation), or viral transduction. The goal is to ensure that the host cells can express the recombinant protein at significant levels.
- Selection and Amplification: Following gene integration, cells that have successfully incorporated the gene of interest must be selected. This process often employs selectable markers, such as antibiotic resistance genes. The use of methotrexate (MTX) amplification technology or the glutamine synthetase (GS) system is common. These systems enhance gene copy number within the host cells, thereby increasing protein expression levels. MTX amplification exploits the dihydrofolate reductase (DHFR) gene, while the GS system uses methionine sulfoximine (MSX) to inhibit the glutamine synthetase enzyme in GS-deficient cells.
- Screening and Cloning: Screening involves evaluating a large number of cell clones to identify those that produce high levels of the target protein. This step is crucial for isolating high-producing clones and is often achieved using sophisticated technologies such as fluorescence-activated cell sorting (FACS) and high-throughput screening systems like ClonePix and Cell Xpress™. These methods enable the identification and isolation of the most productive clones through various fluorescence and imaging techniques.
- Stability Testing: Stability testing is vital to ensure that the selected high-producing clones maintain consistent production over time. This involves evaluating the genetic stability of the clones and their ability to sustain high-level protein production across multiple generations. Stability is crucial for large-scale production and compliance with regulatory standards.
The Cell Line Development Process
Comprehensive Overview of the Cell Line Development Process
The cell line development process is a multi-stage procedure that involves the creation, selection, and optimization of cell lines capable of producing therapeutic proteins. Here is a detailed, integrated overview of the entire cell line development workflow:
- Host Cell Line Selection: The process begins with the selection of an appropriate host cell line. Chinese Hamster Ovary (CHO) cells are the preferred choice due to their adaptability to suspension culture, rapid growth, ability to grow in serum-free media, and capability to produce human-like post-translational modifications. Other mammalian cell lines, such as HEK293, may also be used depending on the specific requirements of the therapeutic protein.
- Gene Transfection and Integration: The gene of interest, which encodes the target protein, is introduced into the host cells using various transfection methods such as electroporation, lipofection, or viral vectors. Efficient gene integration is critical, and this can involve random integration or targeted gene insertion using site-specific recombinases. The goal is to ensure stable and high-level expression of the gene within the host cell genome, whether the protein produced is intended for therapeutic use, research, or other applications.
- Selection and Gene Amplification: After transfection, cells are cultured in selective media containing antibiotics or other agents to ensure only those cells that have successfully integrated the gene of interest survive. Techniques such as methotrexate (MTX) amplification or the glutamine synthetase (GS) system are used to increase the gene copy number, thereby enhancing protein expression. This step involves selecting cells that express the selectable marker alongside the gene of interest.
- Screening and Isolation of High-Producing Clones: The next critical stage is the screening and isolation of clones that produce high levels of the target protein. Techniques like fluorescence-activated cell sorting (FACS), ClonePix, and Cell Xpress™ are employed to identify and isolate high-producing clones. These methods allow for the efficient selection of monoclonal populations derived from a single progenitor cell, ensuring clonality and high productivity.
- Stability Testing: Once high-producing clones are identified, they undergo rigorous stability testing to ensure they maintain consistent protein production over multiple generations. This involves culturing the cells over extended periods and regularly measuring protein expression levels, growth rates, and genetic stability. Stable cell lines are essential for large-scale production and regulatory approval.
- Scale-Up and Bioprocess Optimization: The process is then scaled up from laboratory to industrial bioreactors. Optimization of culture conditions, including media composition, temperature, pH, and feeding strategies, is crucial to maximize protein yield and maintain product quality. This stage often involves iterative adjustments to the bioprocess parameters to achieve the best performance from the selected cell lines.
- Characterization and Validation: The final stage involves comprehensive characterization and validation of the cell lines. This includes assessing the glycosylation patterns, protein structure, and biological activity of the produced protein to ensure it meets all regulatory and therapeutic requirements. Creating a master cell bank of the selected clone is also part of this stage, ensuring a consistent source of cells for future production.
Methods of Gene Transfection in Cell Line Development
Overview of Transfection Methods
Transfection is a crucial step in the cell line development process, as it involves introducing foreign DNA into host cells to enable the expression of the target protein. Various transfection methods are employed depending on the type of host cells, the nature of the DNA, and the desired efficiency of gene integration. Here, we provide an overview of the most commonly used transfection methods, emphasizing their applications in stable cell line development, particularly with CHO cells.
Chemical Transfection
Chemical transfection methods involve using chemical compounds to facilitate the entry of DNA into cells. Common chemical transfection agents include:
- Calcium Phosphate: This method involves mixing DNA with calcium chloride and then adding this mixture to a phosphate buffer. The resulting DNA-calcium phosphate precipitates are then added to the cells. This method is relatively simple and cost-effective but can result in variable transfection efficiency and is often less effective with CHO cells.
- Cationic Lipids (Lipofection): Lipofection uses cationic lipids to form liposomes that encapsulate the DNA. These liposomes fuse with the cell membrane, facilitating DNA entry into the cells. Lipofection is widely used due to its higher efficiency and lower toxicity compared to calcium phosphate transfection. It is particularly effective for transient and stable transfection of CHO cells.
- Polyethylenimine (PEI): PEI is a cationic polymer that binds to DNA, forming complexes that are internalized by the cells. PEI-mediated transfection is efficient and scalable, making it suitable for both small-scale laboratory experiments and large-scale biopharmaceutical production.
Physical Transfection
Physical transfection methods involve the use of physical forces to introduce DNA into cells. Key methods include:
- Electroporation: This method applies an electric field to cells to create temporary pores in the cell membrane through which DNA can enter. Electroporation is highly efficient and can be used for a wide range of cell types, including CHO cells. However, it requires specialized equipment and can be more labor-intensive.
- Microinjection: In microinjection, DNA is directly injected into the nucleus or cytoplasm of individual cells using a fine needle. This method allows precise control over DNA delivery but is technically challenging and time-consuming, making it less practical for high-throughput applications.
- Biolistic Particle Delivery (Gene Gun): This technique uses high-velocity microprojectiles coated with DNA to penetrate the cell membrane and deliver DNA into the cells. It is primarily used in plant and some animal cell applications but is less common in CHO cell line development due to lower efficiency and higher complexity.
Viral Transfection
Viral transfection, or transduction, involves using viral vectors to deliver DNA into host cells. This method leverages the natural ability of viruses to infect cells and introduce their genetic material. Common viral vectors include:
- Adenoviruses: Adenoviral vectors are used for transient transfection due to their high transduction efficiency and ability to infect dividing and non-dividing cells. They are often used in early-stage research but are less suitable for creating stable cell lines.
- Lentiviruses: Lentiviral vectors can integrate into the host genome, making them useful for stable transfection. They can transduce both dividing and non-dividing cells, providing long-term expression of the target gene. Lentiviral transduction is a powerful tool for creating stable CHO cell lines.
- Retroviruses: Retroviral vectors integrate into the host genome but only infect dividing cells. They are commonly used for stable gene expression in a variety of cell types, including CHO cells, although their use is limited by the potential for insertional mutagenesis.
Stable vs. Transient Cell Lines
Definitions and Differences
Stable Cell Lines
Stable cell lines are cell populations that have been genetically modified to integrate a foreign gene permanently into their genome. This genomic integration ensures that the gene is stably inherited by all progeny cells, enabling long-term and consistent expression of the gene product. The development of stable cell lines involves several critical steps: transfection with the gene of interest, selection of successfully transfected cells, and screening for clones that exhibit high and stable expression levels of the target protein over extended periods. This process is rigorous and time-consuming but results in cell lines that are highly valuable for continuous production of recombinant proteins, functional studies, and other applications requiring sustained gene expression.
Transient Cell Lines
Transient cell lines, in contrast, are created by the temporary introduction of a foreign gene into cells without its integration into the host genome. Consequently, the introduced gene is episomal, leading to its expression for a limited duration, typically ranging from a few days to a couple of weeks. As cells divide, the introduced DNA is not replicated or inherited by daughter cells, resulting in a gradual decline in gene expression. Transient transfection is advantageous due to its speed and simplicity, making it suitable for short-term experiments, preliminary protein expression, and rapid testing of genetic constructs. However, the expression levels in transient systems can be variable and decrease over time as the non-integrated DNA is lost during cell division.
Advantages and Disadvantages of Each Type
Type | Advantages | Disadvantages |
---|---|---|
Stable Cell Lines | Consistent Expression: Provide long-term, consistent expression of the gene of interest, essential for large-scale production and reproducibility. | Time-Consuming Development: Involves a lengthy process of transfection, selection, and screening, which can take several months. |
Cost-Effective for Long-Term Use: Once established, can be used repeatedly, reducing the cost per experiment or production batch over time. | Higher Initial Costs: The initial cost of developing stable cell lines is higher due to the need for extensive screening and validation. | |
Genetic Stability: Maintain genetic integrity over successive generations, ensuring reliability and quality in biopharmaceutical production. | Limited Flexibility: Modifying the gene of interest requires restarting the development process. | |
Transient Cell Lines | Quick Setup: Transient transfection can be performed rapidly, with gene expression detectable within a few days. | Variable Expression: Gene expression levels can vary between cells and diminish over time, leading to inconsistency. |
Lower Initial Costs: The process is less resource-intensive than developing stable cell lines, making it more cost-effective for short-term experiments. | Short Duration: The transient nature of gene expression limits the use of these cell lines to short-term studies and applications. | |
Flexibility: Allows for easy testing of multiple constructs or conditions without the need for extensive development. | Not Suitable for Large-Scale Production: Variability and temporary expression make transient cell lines unsuitable for commercial production. |
Applications
Application Type | Stable Cell Lines | Transient Cell Lines |
---|---|---|
Therapeutic Applications | Monoclonal Antibodies (mAbs): Essential for large-scale production, ensuring high-quality and consistent antibodies for treating diseases such as cancer and autoimmune disorders.
Vaccines: Used for consistent and scalable production of viral vaccines, crucial for maintaining supply and rapid response to emerging diseases. Enzyme Replacement Therapies: Required for producing therapeutic enzymes with correct folding and glycosylation for diseases like Gaucher’s and Fabry disease. Hormones and Growth Factors: Necessary for consistent production of hormones like insulin and erythropoietin for treating endocrine disorders and anemia. |
Rapid Protein Production: Ideal for quick production of proteins for preliminary studies and early-stage research.
Feasibility Studies: Used to evaluate gene expression and potential before developing stable cell lines. |
Industrial and Research Applications | Bioprocessing and Biomanufacturing: Crucial for industrial-scale production of biologics, ensuring high yield and quality.
Drug Screening and Toxicology Testing: Used for high-throughput screening and toxicology testing, requiring consistent expression of drug targets. Functional Genomics and Proteomics: Enables long-term studies of gene function and protein interactions, requiring stable expression or knockdown. Diagnostic Applications: Produces diagnostic reagents like antibodies and antigens with high quality and consistency. |
Short-Term Studies: Suitable for testing gene constructs, promoter activity, and protein interactions in short-term experiments.
Synthetic Biology and Metabolic Engineering: Allows quick testing and optimization of genetic circuits or pathways. |
Challenges and Solutions in Cell Line Development
Common Challenges
Developing stable cell lines for biopharmaceutical production is a complex and multi-faceted process that presents several challenges. Understanding and addressing these challenges is crucial for optimizing the cell line development process:
- Genetic Instability: One of the major challenges in the cell line development process is ensuring the genetic stability of the integrated gene. Over time, cells can undergo genetic changes that may lead to a loss or reduction in the expression of the target protein. This is particularly challenging in mammalian cell line development, where maintaining the stable expression of recombinant proteins over many generations is essential.
- Low Productivity: Achieving high levels of protein expression is another significant challenge. Some cell lines may not produce the desired protein in sufficient quantities, which can hinder large-scale biopharmaceutical production. This issue is often encountered in CHO cell line development, where optimizing expression levels is critical for commercial viability.
- Selection of High-Producing Clones: Identifying and isolating high-producing clones from a large population of transfected cells can be time-consuming and labor-intensive. The variability in transfection efficiency and gene integration sites can result in a wide range of expression levels among clones.
- Clone Stability and Consistency: Ensuring that selected clones remain stable and consistently express the target protein over extended periods is crucial. Clonal variability can lead to inconsistencies in protein production, affecting the quality and efficacy of the biopharmaceutical product.
- Regulatory Compliance: Meeting regulatory requirements for cell line development and biopharmaceutical production involves rigorous testing and documentation. Ensuring clonality, genetic stability, and product consistency are essential for regulatory approval, adding complexity to the development process.
Strategies to Overcome Challenges
To address these challenges, several strategies and technological innovations have been developed:
- Advanced Genetic Engineering Techniques: Utilizing advanced genetic engineering tools such as CRISPR/Cas9 can enhance the precision and efficiency of gene integration. This technology allows for targeted modifications to the genome, reducing the likelihood of genetic instability and enhancing the overall productivity of the cell line.
- High-Throughput Screening and Automation: Implementing high-throughput screening methods and automated systems can streamline the identification and isolation of high-producing clones. Technologies such as FACS, ClonePix, and automated imaging systems enable rapid screening of thousands of clones, ensuring the selection of those with the highest productivity and stability.
- Optimization of Culture Conditions: Fine-tuning culture conditions, including media composition, temperature, pH, and feeding strategies, can significantly improve protein yield and cell line stability. Utilizing serum-free and chemically defined media can enhance reproducibility and reduce the risk of contamination.
- Single-Cell Analysis: Employing single-cell RNA sequencing (scRNA-seq) and other single-cell technologies can provide detailed insights into the transcriptional profiles of individual cells. This allows for the identification of rare, high-producing clones and a better understanding of clonal variability, leading to more efficient cell line development workflows.
- Stable Expression Systems: Utilizing stable expression systems, such as the GS and DHFR amplification systems, can enhance gene expression and maintain stability. These systems select for high gene copy numbers and stable integration sites, ensuring consistent and high-level expression of the target protein.
- Rigorous Stability Testing: Conducting thorough stability testing over multiple generations is essential to ensure that selected clones remain genetically stable and consistently produce the target protein. This involves regular monitoring of gene expression levels, growth rates, and protein quality.
- Regulatory Documentation and Compliance: Developing comprehensive documentation and adhering to regulatory guidelines throughout the cell line development process is crucial for achieving regulatory approval. Detailed clonality reports, stability studies, and quality control measures ensure compliance with regulatory standards.
Comparison of Cell Line Development Techniques
Comparative Analysis of Different Techniques
Cell line development involves a variety of techniques, each offering unique advantages and challenges. Here is a concise comparison of traditional and modern methods used in the cell line development process.
Traditional Techniques
- Random Integration
Method: Introduces the gene of interest randomly into the host cell genome using chemical transfection methods like calcium phosphate or lipofection.
Applications: Commonly used in early cell line development.
Efficiency: Variable; random integration can lead to gene silencing or low expression levels due to position effects.
Scalability: Requires extensive screening to identify high-producing clones, which can be time-consuming.
- Gene Amplification Systems
Method: Uses methotrexate (MTX) amplification targeting dihydrofolate reductase (DHFR) or the glutamine synthetase (GS) system to increase gene copy number.
Applications: Widely used in CHO cell line development to enhance protein production.
Efficiency: Can significantly increase expression levels but involves a lengthy selection process.
Scalability: Effective for large-scale production but development can be slow.
Modern Techniques
- CRISPR/Cas9 Genome Editing
Method: Employs CRISPR/Cas9 for precise genome editing.
Applications: Used for targeted gene integration, knockout studies, and enhancing stability and productivity.
Efficiency: High precision and efficiency; reduces off-target effects.
Scalability: Highly scalable with consistent results.
- Site-Specific Integration
Method: Uses site-specific recombinases (e.g., Flp/FRT, Cre/LoxP) for targeted gene integration.
Applications: Ensures consistent expression levels by avoiding position effect variegation.
Efficiency: High; targeted integration leads to predictable and stable gene expression.
Scalability: Suitable for both small-scale research and large-scale production.
- Single-Cell Technologies
Method: Utilizes microfluidics and single-cell RNA sequencing for detailed clone analysis and selection.
Applications: Enhances the accuracy of clone selection.
Efficiency: Allows identification of rare, high-producing clones.
Scalability: Suitable for research and commercial applications due to high-resolution screening capabilities.
Pros and Cons of Different Techniques
Choosing the appropriate technique for cell line development depends on the project’s specific needs, including desired gene expression levels, stability, and scalability. Traditional methods like random integration and gene amplification are useful for certain applications, while modern techniques such as CRISPR/Cas9 and site-specific integration offer greater precision and efficiency. Single-cell technologies further improve clone selection, making them invaluable for both research and commercial biopharmaceutical production. Understanding the pros and cons of each technique allows researchers to optimize their cell line development workflows for the best possible outcomes.
Technique | Pros | Cons |
---|---|---|
Random Integration | Simple and widely used; low initial cost. | Variable gene expression; potential for gene silencing; extensive screening required. |
Gene Amplification Systems | Enhances gene copy number; improves protein production. | Time-consuming; involves multiple rounds of selection and amplification; may lead to genetic instability. |
CRISPR/Cas9 Genome Editing | High precision and efficiency; reduces off-target effects; allows for targeted integration. | Requires advanced expertise and equipment. |
Site-Specific Integration | Consistent expression levels; avoids position effect variegation; predictable and stable gene expression. | Limited to cell lines compatible with recombinase systems; initial setup may be complex. |
Single-Cell Technologies | Provides detailed single-cell analysis; enhances clone selection accuracy; identifies rare, high-producing clones. | High initial cost for equipment and reagents; requires specialized training for operation and data analysis. |
Regulatory Considerations
Compliance with International Standards
In the cell line development process, ensuring compliance with international regulatory standards is crucial for the successful approval and commercialization of biopharmaceutical products. Here are key regulatory frameworks and guidelines that must be adhered to:
- Good Manufacturing Practice (GMP)
- Overview: GMP guidelines are established by regulatory agencies such as the US Food and Drug Administration (FDA), the European Medicines Agency (EMA), and other international bodies. These guidelines ensure that products are consistently produced and controlled according to quality standards.
- Applications: GMP compliance is mandatory for the production of biopharmaceuticals, including those developed using CHO cell line development.
- Requirements: GMP guidelines cover all aspects of production, from raw materials and equipment to the training and hygiene of staff. Ensuring traceability and maintaining detailed records are critical components.
- International Council for Harmonisation (ICH)
- Overview: The ICH provides harmonized guidelines to ensure that biopharmaceutical products meet safety, quality, and efficacy standards across different regions.
- Applications: ICH guidelines, such as ICH Q5D (Derivation and Characterization of Cell Substrates), are particularly relevant to cell line development processes.
- Requirements: These guidelines address the characterization of cell substrates, including testing for adventitious agents and ensuring genetic stability over time.
- World Health Organization (WHO)
- Overview: WHO guidelines provide international standards for the quality, safety, and efficacy of biopharmaceuticals.
- Applications: WHO guidelines are often used by countries to develop their national regulatory frameworks.
- Requirements: WHO guidelines emphasize the importance of comprehensive documentation and validation throughout the cell line development procedure.
- European Pharmacopeia (Ph. Eur.)
- Overview: The European Pharmacopeia sets quality standards for medicines and their components in Europe.
- Applications: Ph. Eur. standards are critical for ensuring that biopharmaceuticals produced using cell lines meet stringent quality criteria.
- Requirements: Includes detailed guidelines on the characterization and testing of cell lines, ensuring they are free from contaminants and genetically stable.
Ethical Considerations
Ethical considerations are paramount in the development of cell lines, particularly when using mammalian cells. These considerations ensure the ethical sourcing and use of biological materials and the welfare of animals involved in the research.
- Informed Consent
- Overview: When human cells are used in cell line development, obtaining informed consent from donors is essential. This ensures that donors are fully aware of how their cells will be used.
- Applications: Informed consent is critical in the derivation of cell lines from human tissues.
- Requirements: Documentation of consent must be thorough and comply with ethical guidelines and regulations.
- Animal Welfare
- Overview: The use of animal cells, such as CHO cells, in cell line development must adhere to ethical standards that ensure the humane treatment of animals.
- Applications: Ethical considerations apply to the sourcing of cells and any in vivo testing required during the development process.
- Requirements: Institutions must follow guidelines such as the 3Rs (Replacement, Reduction, Refinement) to minimize animal use and suffering.
- Biosafety and Bioethics
- Overview: Ensuring biosafety is crucial in the handling and manipulation of cell lines to prevent contamination and ensure the safety of laboratory personnel.
- Applications: Biosafety measures are integral to the cell line development workflow, from initial transfection to large-scale production.
- Requirements: Compliance with biosafety guidelines and regular training for laboratory personnel are mandatory.
- Intellectual Property (IP) Rights
- Overview: Protecting the intellectual property rights associated with cell line development is essential for innovation and commercialization.
- Applications: IP rights cover the proprietary methods, cell lines, and products developed during the cell line development process.
- Requirements: Proper documentation and legal protections must be in place to safeguard proprietary technologies and cell lines.
FAQs and Common Questions
- How do you select the best host cell line for cell line development? The selection of the host cell line depends on the specific requirements of the protein being produced. CHO cells are commonly selected for their adaptability to suspension culture, high productivity, and ability to produce human-like glycosylation patterns. Other mammalian cells like HEK293 may be used for certain applications where specific post-translational modifications are needed.
- What criteria are used to identify high-producing clones? High-producing clones are identified based on several criteria, including the level of protein expression, growth rate, genetic stability, and the ability to maintain consistent production over time. Advanced screening technologies such as FACS and ClonePix are used to evaluate these factors.
- How does the use of serum-free media benefit the cell line development process? Serum-free media eliminates the variability associated with serum-containing media, providing a more controlled and consistent environment for cell growth. It reduces the risk of contamination and simplifies downstream processing. Additionally, serum-free conditions are more aligned with regulatory requirements for therapeutic protein production.
- What are the common pitfalls in cell line development and how can they be avoided? Common pitfalls include genetic instability, low productivity, and contamination. These can be avoided by using advanced genetic engineering techniques like CRISPR/Cas9, employing rigorous selection and screening processes, optimizing culture conditions, and maintaining strict biosafety protocols.
- How does ProteoGenix ensure high productivity in stable cell line development? ProteoGenix leverages its advanced Verified In-Situ Plate Seeding (VIPS™) technology, which ensures high-efficiency single-cell seeding with image-based proof of clonality. This technology accelerates development times by more than 50%, ensuring robust productivity. Additionally, ProteoGenix uses proprietary, IP-free cell lines and serum-free media to enhance productivity and provide strong yield guarantees.
- What are the unique advantages of ProteoGenix’s stable cell line generation service? ProteoGenix offers several unique advantages, including the use of VIPS™ technology for high-efficiency cloning, image-based proof of clonality, and regulatory confidence with detailed clonality reports. The service is also cost-effective with no royalties, and it ensures flexibility and customization to meet specific project needs. Clients retain IP rights over the developed cell lines, simplifying protocol transfer and large-scale production.
- What are ProteoGenix’s recommendations for maximizing production yields? ProteoGenix recommends extensive early testing of antibody leads before large-scale production. This includes in silico developability assessment and early stage characterization to identify the most stable and effective sequences. This approach helps bypass expression hurdles and ensures optimal production at reasonable costs. They also emphasize using CHO cells for their ability to produce human-like glycosylation and higher tolerance to environmental changes.
Conclusion
Cell line development is a pivotal aspect of biopharmaceutical production, encompassing a comprehensive workflow that ensures the creation of genetically stable and highly productive cell lines. This intricate process involves selecting suitable host cell lines, employing advanced gene transfection techniques, and utilizing high-throughput screening and automation to identify high-producing clones. With significant advancements in genetic engineering, such as CRISPR/Cas9 and single-cell technologies, the efficiency and precision of cell line development have dramatically improved.
The stability and scalability of the developed cell lines are paramount for the production of therapeutic proteins, monoclonal antibodies, and other biologics. Compliance with international regulatory standards, including GMP, ICH, WHO, and Ph. Eur., ensures that the biopharmaceutical products meet the highest quality, safety, and efficacy standards.
At ProteoGenix, we leverage cutting-edge VIPS™ technology to revolutionize cell line development. Our approach not only accelerates the development timeline by more than 50% but also guarantees high-efficiency single-cell seeding with image-based proof of clonality. This robust and efficient methodology enhances productivity and provides strong yield guarantees without the burden of royalties.
By choosing ProteoGenix, you benefit from our extensive experience, proprietary technologies, and client-centric services tailored to meet your specific project needs. We offer IP-free cell lines, comprehensive stability studies, and detailed regulatory documentation, ensuring a smooth transition from research to large-scale production.
Ready to elevate your monoclonal antibody production and biopharmaceutical research? Partner with ProteoGenix for advanced stable cell line development services. Visit our dedicated service pages to learn more:
- Stable Cell Line Generation Service
- Mammalian Cell Expression Systems
- High-Throughput Antibody Production
Experience the future of biopharmaceutical production with ProteoGenix. Our proven expertise, innovative technologies, and commitment to quality will help you achieve outstanding results in your cell line development projects.