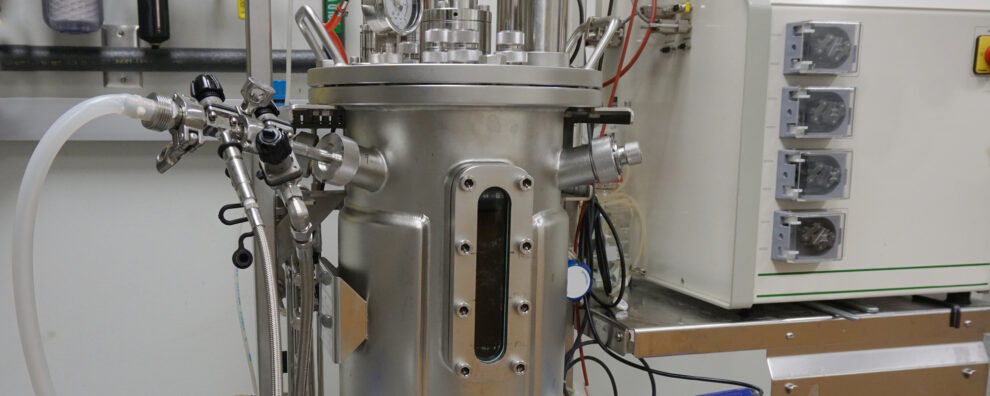
Antibody production: a complete guide
Antibodies play a crucial role in the immune system, identifying and neutralizing foreign substances such as bacteria and viruses. Their unique properties inspired researchers to exploit them in biomedicine, and they are now widely used in diagnostic and therapeutic applications. These applications have been made possible thanks to the development of advanced antibody generation and production techniques. This article aims to describe the fundamentals of antibody production, revisiting the concepts of monoclonal and polyclonal antibodies, detailing the methods of antibody generation and production, and exploring the applications, challenges, and future perspectives of these biomolecules.
- What is antibody production?
- In Vivo Antibody Production
- Antibody production as a biotechnological process
- Recombinant Antibody Production
- Polyclonal Antibody Production
- What are polyclonal antibodies?
- Animals Commonly Used for Polyclonal Antibody Production
- Differences between polyclonal and monoclonal antibodies
- Monoclonal antibody generation
- What are monoclonal antibodies?
- In Vivo Monoclonal Antibody Generation Methods
- Hybridoma Technology
- Single B Cell Sorting
- In Vitro Monoclonal Antibody Generation Methods
- Phage Display Technology
- Monoclonal antibody production methods
- Ascites Production
- Hybridoma Cell Culture
- Recombinant Antibody Production
- What is Recombinant Antibody Production?
- Transient and Stable Antibody Expression
- Transient Antibody Expression
- Stable Antibody Expression
- Expression Systems for Antibody Production
- Bacterial Expression Systems
- Mammalian Expression Systems
- Applications of monoclonal antibodies
- The use of monoclonal antibodies as therapeutics
- Cancer
- Common Cancer Targets
- Autoimmune Diseases
- Common Autoimmune diseases Targets
- Infectious Diseases
- Common Targets
- Diagnostics
- Research
- Challenges and Solutions in Antibody Production
- Immunogenicity
- Low Yield, High Production Costs
- Post-Translational Modifications
- Immunogenic Glycosylation Profiles
- Aggregation and Stability
- Perspectives and Future Trends in Antibody Production
- Integration of Artificial Intelligence
- Advancements in Synthetic Biology
- Novel Expression Systems
- Antibody production at ProteoGenix
- Antibody production FAQ
- What stimulates antibody production?
- How are antibodies produced?
- What are the different steps of an antibody production process?
- Selected publications
What is antibody production?
Antibody production is a complex and multifaceted process, crucial in both natural immunity and therapeutic applications. Understanding this process requires exploring different contexts in which antibodies are generated. Here, we delineate three distinct definitions of antibody production: in vivo antibody production, antibody production seen as a biotechnological process, and recombinant antibody production.
In Vivo Antibody Production
In vivo antibody production refers to the natural process by which an organism’s immune system generates antibodies in response to the presence of antigens. When the body encounters a pathogen, such as a virus or bacterium, the immune system recognizes foreign molecules (antigens) on the pathogen’s surface. This recognition triggers a complex immune response involving various types of immune cells.
Key steps in in vivo antibody production include:
- Antigen Presentation: Dendritic cells capture antigens and present them to T-helper cells.
- B Cell Activation: T-helper cells activate B cells that are specific to the antigen.
- Antibody Secretion: Activated B cells differentiate into plasma cells, which secrete large quantities of antibodies specific to the antigen.
- Memory Formation: Some B cells become memory cells, providing long-term immunity.
This natural process is fundamental to the body’s defense mechanism, providing both immediate and lasting protection against infections.
To leverage the specificity and potency of the immune response, scientists have developed methods to mimic this process in the laboratory. These methods enable the production of antibodies for various applications, including therapeutic, diagnostic, and research purposes. Unlike polyclonal antibodies, which are typically produced in living organisms and consist of a mixture of antibodies targeting different epitopes, monoclonal antibodies can be produced in vitro. This means monoclonal antibody production can be achieved without the need for animals, offering a more controlled and ethical approach.
Antibody production as a biotechnological process
The second definition of antibody production encompasses a comprehensive biotechnological process, starting from antigen preparation and extending to the production of antibodies. This industrial-scale process involves multiple stages, each critical to ensuring the quality and efficacy of the produced antibodies.
This approach includes antigen preparation, screening and selection of high-affinity antibodies, cloning and expression in suitable host cells, and subsequent purification and characterization of the antibodies. It integrates various methods, such as hybridoma technology, phage display, and single B cell sorting, to generate specific antibodies tailored for therapeutic applications.
Recombinant Antibody Production
Recombinant antibody production refers to the generation of antibodies through recombinant DNA technology. This method involves genetically engineering host cells to produce specific antibodies, bypassing the need for immunization in animals.
Steps in recombinant antibody production include:
- Gene Isolation and Cloning: Isolating the genes encoding the desired antibody’s heavy and light chains and inserting them into expression vectors.
- Expression in Host Cells: Introducing these vectors into host cells (e.g., bacteria, yeast, or mammalian cells) to produce the antibody proteins.
- Culturing and Harvesting: Culturing the host cells in bioreactors to express the antibodies, followed by harvesting the culture medium.
- Purification: Purifying the recombinant antibodies to obtain high-quality products suitable for therapeutic use.
Recombinant production allows for greater control over antibody characteristics and enables large-scale manufacturing, making it a preferred method for producing monoclonal antibodies and other therapeutic formats.
Polyclonal Antibody Production
Polyclonal antibody production is a crucial technique in immunology and biotechnology, used for a wide range of applications, including diagnostics and research. This section covers the definition of polyclonal antibodies and the animals commonly used for their production.
What are polyclonal antibodies?
Polyclonal antibodies (pAbs) are a heterogeneous mixture of antibodies produced by different B cell clones in an animal. These antibodies recognize and bind to multiple epitopes on a single antigen, making them highly versatile and effective for detecting various forms of the target antigen.
Animals Commonly Used for Polyclonal Antibody Production
Several animals are used for producing polyclonal antibodies, with the choice depending on the required quantity and specific characteristics of the antibodies. The most commonly used animals include:
- Rabbits: Popular for their relatively large blood volume and ease of handling. They produce a robust immune response, yielding high-affinity antibodies, ideal for diagnostic applications.
- Mice and Rats: Often used for generating small quantities of antibodies. They are suitable for preliminary studies and specific research applications.
- Goats and Sheep: Used for large-scale antibody production due to their larger blood volumes and capacity to generate substantial quantities of antibodies.
- Chickens: An alternative source, especially useful when mammalian antibodies may cross-react with mammalian antigens. They produce antibodies in egg yolk, known as IgY.
Differences between polyclonal and monoclonal antibodies
Understanding the distinctions between polyclonal and monoclonal antibodies is essential to appreciate their respective advantages and applications in research, diagnostics, and therapeutics.
Polyclonal antibodies (pAbs) are produced by multiple B cell clones, resulting in a heterogeneous mixture of antibodies that recognize and bind to various epitopes on a single antigen. This polyclonal nature makes them highly versatile and capable of detecting different forms and variations of the target antigen, which can be particularly useful in applications where a broad detection range is desired. Polyclonal antibodies are also particularly appreciated for the low detection limit, making them useful for various diagnostic and research applications. However, their heterogeneity can also lead to batch-to-batch variability and potential cross-reactivity with non-target antigens.
In contrast, monoclonal antibodies (mAbs) are produced by a single B cell clone, resulting in a homogeneous population of antibodies that recognize and bind to a single, specific epitope on the antigen. This specificity makes monoclonal antibodies highly precise tools for targeting and neutralizing specific molecules. The consistent and reproducible nature of monoclonal antibodies makes them ideal for therapeutic applications, where uniformity and high specificity are critical. Additionally, monoclonal antibodies are less likely to exhibit cross-reactivity compared to polyclonal antibodies.
With these distinctions in mind, we can explore the process of producing monoclonal antibodies, highlighting their unique properties and the sophisticated techniques used to generate them.
Polyclonal Antibodies (pAbs) | Monoclonal Antibodies (mAbs) | |
---|---|---|
Production Source | Produced by multiple B cell clones | Produced by a single B cell clone |
Heterogeneity | Heterogeneous mixture, recognizes various epitopes on a single antigen | Homogeneous, recognizes a single specific epitope on the antigen |
Versatility | Highly versatile, capable of detecting different forms and variations of the target antigen | Highly specific, precise targeting and neutralization of specific molecules |
Detection Range | Broad detection range, useful in applications requiring detection of different antigen forms and variations | Narrow detection range, focused on a single epitope |
Detection Limit | Low detection limit, advantageous for various diagnostic and research applications | Higher detection limit compared to polyclonal antibodies |
Batch Consistency | Potential batch-to-batch variability due to heterogeneous nature | High consistency and reproducibility between batches due to homogeneous nature |
Cross-reactivity | Potential for cross-reactivity with non-target antigens | Less likely to exhibit cross-reactivity |
Therapeutic Suitability | Not suitable for therapeutic applications because of reproducibility | Ideal for therapeutic applications, requiring high specificity and reproducibility |
Production Complexity | Easy and fast to produce | More complex and time-consuming to produce |
Cost | – | + |
Application Suitability | Useful in diagnostic and research applications requiring broad antigen detection and low detection limits | Suitable for therapeutic applications and research requiring precise targeting and consistent results |
Monoclonal antibody generation
Monoclonal antibodies (mAbs) have revolutionized biotechnology, diagnostics, and therapeutics due to their high specificity and uniformity. This section covers the definition of monoclonal antibodies, methods for their production, and the advantages and limitations of each method.
What are monoclonal antibodies?
Monoclonal antibodies are homogeneous antibodies produced by a single clone of B cells. Unlike polyclonal antibodies, which are a mixture of antibodies from different B cells, monoclonal antibodies recognize and bind to a single specific epitope on an antigen. This high specificity makes monoclonal antibodies highly precise tools for targeting specific molecules, providing several key advantages:
- Specificity: Monoclonal antibodies bind to one specific epitope on an antigen, reducing the risk of cross-reactivity and non-specific binding.
- Consistency: As they are produced from a single clone of cells, monoclonal antibodies provide uniformity in their structure and function, ensuring consistent performance across different batches.
- Versatility: They can be engineered and modified for various applications, including diagnostic tests, therapeutic treatments, and research tools.
- Therapeutic Potential: Due to their ability to specifically target disease-related molecules, monoclonal antibodies are widely used in treating conditions such as cancer, autoimmune diseases, and infectious diseases.
In Vivo Monoclonal Antibody Generation Methods
Hybridoma Technology
Hybridoma technology is an in vivo method for producing monoclonal antibodies by fusing antibody-producing B cells from an immunized animal with myeloma cells to create hybrid cells that can be cloned and expanded to produce large quantities of identical antibodies.
Hybridoma technology offers several advantages. The antibodies produced through this method exhibit high specificity and affinity. Additionally, hybridoma cell lines are stable, enabling long-term production of antibodies. The technique itself is well-characterized and widely used in the field.
However, hybridoma technology also has its limitations. The process is time-consuming and labor-intensive, which can be a significant drawback. Moreover, it requires the use of animals, which raises ethical concerns. The method is generally limited to producing antibodies from the species used for immunization, typically mice or rats. Human antibodies can only be obtained by using transgenic mice or through a lengthy humanization process. Furthermore, there is a potential for myeloma cell contamination, which can affect the quality of the antibodies produced.
Single B Cell Sorting
Single B cell sorting is an in vivo method for producing monoclonal antibodies by isolating and cloning individual B cells that produce specific antibodies. These B cells are typically obtained from immunized animals or humans and are sorted based on their antigen specificity.
Single B cell sorting has several advantages. The method produces antibodies with high specificity and affinity. It allows for the rapid identification and cloning of antigen-specific B cells, which is particularly useful for quickly generating antibodies. Additionally, it is suitable for producing human antibodies directly from human donors, avoiding the need for lengthy humanization processes.
However, this method also has limitations. It requires access to immunized individuals or animals, which can be a logistical challenge. The process is technically demanding and requires specialized equipment and expertise. Furthermore, the number of antigen-specific B cells that can be isolated is limited, which can constrain the production scale. When human antibodies are produced from animal sources, they may still require humanization to be fully compatible for therapeutic use.
In Vitro Monoclonal Antibody Generation Methods
Phage Display Technology
Phage display is an in vitro technique for producing monoclonal antibodies by displaying antibody fragments on the surface of bacteriophages (viruses that infect bacteria). This allows for the rapid screening and selection of high-affinity antibodies from large libraries of antibody fragments.
Phage display technology offers several advantages. It does not require animal use, making it an ethical alternative for antibody production. The method enables the rapid generation and screening of large antibody libraries, which significantly speeds up the discovery process. Phage display also provides high flexibility in engineering and modifying antibodies, allowing researchers to tailor antibodies for specific needs. There is no species restriction, meaning antibodies can be derived from any species, which facilitates the generation of fully human antibodies without the need for humanization. Additionally, phage display is highly adaptable for various applications, including the development of antibodies against toxic or non-immunogenic antigens.
However, this method also has its limitations. It requires sophisticated laboratory infrastructure and expertise, which can be a barrier for some research facilities. The antibodies produced may have lower affinity compared to those generated in vivo, which can affect their efficacy. Moreover, the complexity of antigens that can be used in the display system is limited, which can restrict the range of antibodies that can be developed using this technique.
Monoclonal antibody production methods
Ascites Production
Ascites production is an in vivo method that involves injecting hybridoma cells into the peritoneal cavity of a mouse. The hybridoma cells produce antibodies that accumulate in the ascitic fluid, which is then harvested.
One of the primary advantages of ascites production is the high yield of antibodies it can produce in a relatively short period. This method can be particularly useful when large quantities of antibodies are required quickly. Additionally, the antibodies produced in ascites fluid often have high titers and can be readily purified.
However, ascites production has significant drawbacks. The primary concern is ethical, as the method involves the use of live animals, raising concerns about animal welfare. Furthermore, the process can result in variability in antibody quality and yield, depending on the health and condition of the animals used. There is also a risk of contamination with mouse proteins and other unwanted substances, which can complicate the purification process and potentially affect the application of the antibodies in clinical or diagnostic settings.
Hybridoma Cell Culture
Hybridoma cell culture is an in vitro method where hybridoma cells, created by fusing B cells from an immunized mouse with myeloma cells, are cultured in a controlled environment to produce antibodies. The hybridoma cells can be grown in various culture systems, including flasks, bioreactors, or other large-scale production systems.
The major advantage of hybridoma cell culture is the ability to produce monoclonal antibodies with high specificity and affinity in a consistent and controlled manner. Once a stable hybridoma cell line is established, it can be maintained and expanded indefinitely, providing a continuous and reliable source of antibodies. This method also eliminates many of the ethical concerns associated with animal use in ascites production.
Despite its advantages, hybridoma cell culture has its own set of challenges. The process of creating and selecting stable hybridoma cell lines is labor-intensive and time-consuming, often requiring several months to complete. Additionally, hybridoma cells are prone to genetic instability over time, which can result in changes in antibody production and quality. Maintaining hybridoma cultures also requires significant resources, including specialized equipment and expertise, which can be cost-prohibitive for some laboratories.
Recombinant Antibody Production
Recombinant antibody production using DNA technology has become a cornerstone in the field of biopharmaceuticals, offering precise and scalable methods to generate monoclonal antibodies. This section includes a definition of recombinant antibody production, descriptions of transient and stable antibody expression, and an overview of commonly used expression systems.
What is Recombinant Antibody Production?
Recombinant antibody production involves the use of genetic engineering to create antibodies in host cells. This process entails inserting the genes encoding the desired antibody into an expression system, which then produces the antibody. This method allows for high specificity and control over antibody characteristics, making it highly suitable for therapeutic, diagnostic, and research applications. Recombinant technology enables the production of antibodies with consistent quality and the possibility of tailoring antibody features such as affinity and specificity.
Transient and Stable Antibody Expression
In the context of recombinant antibody production, there are two primary approaches: transient and stable expression.
Transient Antibody Expression
Transient expression involves the temporary introduction of plasmid DNA encoding the antibody into host cells. The cells produce the antibody for a short period before the plasmid is lost or degraded. The process begins with the transfection of plasmid DNA into host cells, typically using chemical, physical, or viral methods to introduce the DNA. Once inside the host cells, the plasmid DNA is expressed, and the cells produce the antibody for a limited duration, usually a few days to weeks. During this time, the antibodies are harvested from the cell culture supernatant.
The advantages of transient expression include its rapid production timeline, making it ideal for short-term studies and early-stage research. It is also suitable for producing small to moderate quantities of antibodies without the need for creating stable cell lines. However, the primary limitations include the transient nature of expression, requiring frequent transfections for sustained production, and generally lower yield and consistency compared to stable expression systems.
Stable Antibody Expression
Stable expression involves integrating the antibody genes into the host cell genome, allowing for long-term and continuous production of antibodies. This process starts with the transfection of plasmid DNA into host cells. Cells that have integrated the antibody genes into their genome are then selected using antibiotic resistance markers. These selected cells are cloned to establish stable cell lines that consistently express the antibody. Once established, these cell lines can produce antibodies continuously over an extended period.
The advantages of stable expression include long-term and consistent production, high yield, and scalability, making it suitable for large-scale manufacturing. Unlike transient expression, stable cell lines reduce the need for repeated transfections, offering a more efficient and reliable production process. However, establishing stable cell lines is time-consuming and labor-intensive, requiring a complex and costly initial setup.
Transient Antibody Expression | Stable Antibody Expression | |
---|---|---|
Expression Method | Temporary introduction of plasmid DNA into host cells | Integration of antibody genes into the host cell genome |
Duration | Short-term (few days to weeks) | Long-term and continuous |
Production Timeline | Rapid production, suitable for short-term studies and early-stage research | Time-consuming initial setup |
Quantity Produced | Small to moderate quantities | High yield and scalability |
Applications | Early-stage research, proof-of-concept studies, small-scale production | Large-scale manufacturing, therapeutic antibody production, consistent and reliable antibody supply |
Advantages | Rapid production timeline; suitable for producing small to moderate quantities without stable cell lines | Long-term consistent production; high yield; scalable for large-scale manufacturing |
Limitations | Transient nature of expression; lower yield and consistency compared to stable systems | Time-consuming and labor-intensive to establish; requires a complex and costly initial setup |
Expression Systems for Antibody Production
Several expression systems are used for recombinant antibody production, each with distinct advantages and limitations. The most commonly used systems include bacterial and mammalian expression systems.
Bacterial Expression Systems
Bacterial systems, particularly Escherichia coli (E. coli) and Bacillus subtilis, are popular for antibody production due to their rapid growth and high-density cultures, leading to high productivity. The cultivation process is cost-effective and straightforward, with well-characterized genetics and a wide range of molecular biology tools available.
However, bacterial systems have limitations in producing full-length antibodies with proper post-translational modifications, such as glycosylation, which are essential for the functionality and stability of therapeutic antibodies. As a result, bacterial systems are primarily used for producing smaller antibody fragments like single-chain variable fragments (scFvs) and fragment antigen-binding (Fab) fragments. These fragments are suitable for research, diagnostics, and certain therapeutic applications where full-length antibodies are not required. Additionally, protein folding and solubility issues can arise with complex antibody structures in bacterial systems.
Mammalian Expression Systems
Mammalian systems, particularly Chinese Hamster Ovary (CHO) cells and Human Embryonic Kidney (HEK) 293 cells, are widely used for antibody production due to their ability to produce full-length antibodies with proper post-translational modifications, including glycosylation. These modifications are crucial for the function and stability of therapeutic antibodies. Mammalian systems also achieve high expression levels and scalability, particularly in CHO cells, making them suitable for large-scale manufacturing.
Mammalian systems are more complex and costly to cultivate compared to bacterial systems, with a longer development time required to establish stable cell lines. They also require more sophisticated infrastructure and expertise. Despite these challenges, mammalian systems are essential for producing a wide range of antibody formats, including full-length IgG antibodies, bispecific antibodies, and Fc-fusion proteins.
Bacterial Expression Systems | Mammalian Expression Systems | |
---|---|---|
Growth and Cultivation | Rapid growth and high-density cultures | More complex to cultivate |
Productivity | High productivity | High expression levels and scalability |
Cost | Cost-effective and straightforward | More costly |
Genetic Tools | Well-characterized genetics and wide range of molecular biology tools available | Requires more sophisticated genetic tools |
Antibody Types Produced | Primarily smaller antibody fragments like scFvs and Fab fragments | Full-length IgG antibodies, bispecific antibodies, and Fc-fusion proteins |
Post-Translational Modifications | Limited ability for proper post-translational modifications such as glycosylation | Capable of proper post-translational modifications, including glycosylation |
Protein Folding/Solubility | Protein folding and solubility issues with complex antibody structures | Better handling of protein folding and solubility |
Applications | Suitable for research, diagnostics, and certain therapeutic applications | Suitable for therapeutic applications requiring full-length antibodies |
Recombinant antibody production using DNA technology is a versatile and scalable approach for generating monoclonal antibodies. The choice between transient and stable expression methods depends on the desired production scale and timeline. Bacterial systems are ideal for producing smaller antibody fragments quickly and cost-effectively, while mammalian systems are necessary for producing fully functional antibodies with the appropriate modifications for therapeutic use. Understanding the advantages and limitations of each method and system enables researchers and manufacturers to select the most suitable approach based on their specific requirements.
Applications of monoclonal antibodies
Antibody production has revolutionized various fields, particularly in therapeutics, diagnostics, and research. Each application leverages the unique properties of antibodies to achieve specific goals, from treating diseases to developing diagnostic tools and advancing scientific understanding.
The use of monoclonal antibodies as therapeutics
In therapeutics, monoclonal antibodies (mAbs) are employed to treat a wide range of diseases, including cancers, autoimmune disorders, and infectious diseases. Therapeutic antibodies can be designed to target specific antigens on cancer cells, marking them for destruction by the immune system or directly inhibiting their growth. Examples include rituximab for lymphoma and trastuzumab for breast cancer. Additionally, antibodies are used to modulate immune responses in conditions like rheumatoid arthritis and multiple sclerosis by targeting specific cytokines or cell surface receptors.
One of the significant challenges in therapeutic antibody production is the risk of immunogenicity, where the patient’s immune system recognizes the antibody as foreign and mounts an immune response against it. This issue can be mitigated by using humanized or fully human antibodies produced through transgenic mice or human phage display libraries. Another challenge is achieving high yield and purity, which is crucial for therapeutic applications. Solutions include optimizing cell culture conditions, using high-efficiency expression systems, and employing advanced purification techniques.
Cancer
Monoclonal antibodies have been transformative in oncology, offering targeted treatments that can specifically bind to cancer cells while sparing normal tissues. Unlike classical chemotherapy, which often affects both cancerous and healthy cells, leading to significant side effects, monoclonal antibodies provide a more precise approach. Commonly targeted cancers include breast cancer, lymphoma, leukemia, and colorectal cancer.
Common Cancer Targets
HER2/neu: Trastuzumab (Herceptin) targets the HER2/neu receptor, which is overexpressed in some breast cancers. By binding to this receptor, trastuzumab inhibits the proliferation of cancer cells and induces antibody-dependent cellular cytotoxicity (ADCC).
CD20: Rituximab (Rituxan) targets CD20, a protein expressed on the surface of B-cells. This antibody is used to treat non-Hodgkin lymphoma and chronic lymphocytic leukemia (CLL) by inducing cell lysis through ADCC and complement-dependent cytotoxicity (CDC).
EGFR: Cetuximab (Erbitux) targets the epidermal growth factor receptor (EGFR), which is overexpressed in various cancers, including colorectal cancer and head and neck cancers. By blocking EGFR signaling, cetuximab inhibits tumor growth and proliferation.
VEGF: Bevacizumab (Avastin) targets vascular endothelial growth factor (VEGF), a key regulator of angiogenesis. By inhibiting VEGF, bevacizumab reduces the blood supply to tumors, thereby inhibiting their growth.
Autoimmune Diseases
Monoclonal antibodies are used to treat autoimmune diseases by targeting specific components of the immune system that are involved in the pathological immune response. Commonly treated conditions include rheumatoid arthritis, multiple sclerosis, and psoriasis.
Common Autoimmune diseases Targets
TNF-α: Infliximab (Remicade) and adalimumab (Humira) target tumor necrosis factor-alpha (TNF-α), a cytokine involved in systemic inflammation. By neutralizing TNF-α, these antibodies reduce inflammation and halt disease progression in rheumatoid arthritis, psoriasis, and inflammatory bowel disease (IBD).
IL-6 Receptor: Tocilizumab (Actemra) targets the interleukin-6 (IL-6) receptor, blocking IL-6 signaling. This action is beneficial in treating rheumatoid arthritis by reducing inflammation and joint damage.
CD20: Ocrelizumab (Ocrevus) targets CD20 and is used in the treatment of multiple sclerosis. By depleting B-cells, it helps in reducing disease activity and progression.
Infectious Diseases
Monoclonal antibodies have also shown promise in the treatment and prevention of infectious diseases. They can provide passive immunity by targeting specific pathogens or their toxins.
Common Targets
RSV F Protein: Palivizumab (Synagis) targets the fusion protein of the respiratory syncytial virus (RSV). It is used as a prophylactic treatment to prevent RSV infection in high-risk infants.
Clostridium difficile Toxin B: Bezlotoxumab (Zinplava) targets toxin B produced by Clostridium difficile, reducing the recurrence of C. difficile infections by neutralizing the toxin.
Diagnostics
Antibodies are indispensable tools in diagnostics due to their high specificity and affinity for their target antigens. They are used in various diagnostic assays, such as enzyme-linked immunosorbent assays (ELISA), lateral flow assays (such as pregnancy tests), and immunohistochemistry. These assays can detect and quantify specific proteins, pathogens, or biomarkers in biological samples, aiding in disease diagnosis, monitoring, and research.
A common challenge in diagnostic antibody production is ensuring batch-to-batch consistency and high sensitivity. This can be addressed by using recombinant antibody production methods, which provide greater control over antibody characteristics and reproducibility. Additionally, antibodies used in diagnostics must exhibit high stability and resistance to environmental variations, which can be improved through protein engineering and selection of robust antibody formats.
Research
In research, antibodies are essential for a variety of applications, including Western blotting, flow cytometry, immunoprecipitation, and microscopy. They allow scientists to study the presence, quantity, and function of proteins within complex biological systems, contributing to our understanding of cellular processes and disease mechanisms.
Research applications often require antibodies with specific characteristics, such as high affinity and minimal cross-reactivity. Producing such antibodies can be challenging, especially for targets that are highly conserved or poorly immunogenic. Hybridoma technology and phage display libraries offer solutions by enabling the selection of high-affinity antibodies against a wide range of antigens. Additionally, recombinant antibody technologies allow for the rapid production and modification of antibodies to meet specific research needs.
Challenges and Solutions in Antibody Production
The production of monoclonal antibodies, while highly beneficial, presents several technical and logistical challenges. These include immunogenicity, low yield, post-translational modifications, immunogenic glycosylation profiles, aggregation, and stability.
Immunogenicity
Therapeutic antibodies can sometimes trigger immune responses in patients, particularly when they contain non-human sequences. This challenge is addressed by using humanized or fully human antibodies. Humanization involves modifying a non-human antibody (typically derived from a mouse) to make its protein sequence more similar to human antibodies. This is done by grafting the non-human antibody’s complementarity-determining regions (CDRs), which are responsible for antigen binding, onto a human antibody framework. Additionally, transgenic mice that express human immunoglobulin genes and human phage display libraries enable the production of antibodies with minimal immunogenicity, reducing the likelihood of adverse immune reactions. These approaches help produce therapeutic antibodies that are less likely to be recognized as foreign by the patient’s immune system, thereby reducing the risk of immunogenicity.
Low Yield, High Production Costs
Achieving high yields of functional antibodies is critical, especially for therapeutic applications. Low yields directly contribute to high production costs, as more resources and time are required to produce sufficient quantities of the antibody. This challenge can be addressed by optimizing cell culture conditions, such as nutrient composition, temperature, and pH. Advances in bioreactor technology and the use of high-efficiency expression systems, such as CHO cells, also contribute to increased antibody production, thus helping to reduce costs.
Another critical aspect is antibody developability, which refers to the ease with which an antibody can be developed into a stable, manufacturable, and effective therapeutic product. Factors influencing developability include the antibody’s stability, solubility, and expression levels in production systems. Screening antibodies early in the development process for properties such as high expression levels, stability under manufacturing conditions, and minimal aggregation can enhance developability. By focusing on these characteristics, manufacturers can identify and prioritize antibody candidates that are more likely to yield high quantities and maintain their functional integrity, ultimately reducing production costs and improving the efficiency of the development pipeline.
Post-Translational Modifications
Proper post-translational modifications, including glycosylation, are essential for the stability and function of antibodies. Mammalian expression systems are preferred for producing antibodies with human-like glycosylation patterns. However, even in these systems, glycosylation profiles can vary, potentially affecting the efficacy and safety of therapeutic antibodies. Engineering cell lines to produce consistent glycosylation patterns and using glycoengineering techniques can help address this issue.
Immunogenic Glycosylation Profiles
Non-human glycosylation profiles can trigger immune responses in patients. This challenge is particularly relevant when using non-mammalian expression systems. To overcome this, antibodies are often produced in mammalian cells, which are more likely to perform human-like glycosylation. Additionally, glycoengineering techniques can be employed to modify glycosylation patterns and reduce immunogenicity.
Aggregation and Stability
Antibody aggregation can lead to reduced efficacy and increased immunogenicity. Ensuring the stability of antibodies during production, purification, and storage is crucial. This can be achieved by optimizing production conditions, using stabilizing agents, and employing protein engineering techniques to enhance the inherent stability of antibodies. Advanced analytical methods are also used to monitor and control aggregation throughout the production process.
The production of monoclonal antibodies is integral to advancements in therapeutics, diagnostics, and research. Each application benefits from the unique properties of antibodies, but the production process is fraught with challenges. Innovations in genetic engineering, cell culture optimization, and protein engineering offer solutions to these challenges, enabling the development of high-quality antibodies with specific, consistent, and functional properties. Understanding and addressing these challenges is essential for the continued success and expansion of antibody-based applications.
Perspectives and Future Trends in Antibody Production
Integration of Artificial Intelligence
Artificial intelligence is set to revolutionize antibody production by enhancing various aspects of the development process. AI algorithms can analyze vast datasets to identify optimal antibody candidates with high affinity, specificity, and developability. Machine learning models can predict the most promising antibodies based on their sequences, structures, and binding properties, significantly speeding up the discovery phase.
AI can also optimize cell culture conditions and bioprocess parameters. By analyzing real-time data from bioreactors, AI systems can adjust variables such as nutrient composition, temperature, and pH to maximize yield and ensure consistent quality. Furthermore, AI-driven predictive modeling can forecast potential issues in production and suggest preventive measures, reducing downtime and improving overall efficiency.
In post-production, AI tools can aid in the detailed analysis of antibody properties, such as stability and aggregation tendencies.
Advancements in Synthetic Biology
Synthetic biology offers new possibilities for the production of monoclonal antibodies. Techniques such as CRISPR-Cas9 gene editing allow precise modifications to be made to cell lines, enhancing their productivity and ensuring consistent post-translational modifications. Synthetic biology also enables the design of entirely new antibody formats, such as bispecific antibodies and antibody-drug conjugates (ADCs), which can target multiple antigens or deliver therapeutic payloads directly to disease sites.
Engineered microbes and yeast are being developed as alternative expression systems that can produce antibodies with human-like glycosylation patterns. These systems promise to reduce production costs and increase scalability, making antibody therapies more accessible.
Novel Expression Systems
The development of novel expression systems is another exciting trend in antibody production. Mammalian cells, particularly CHO cells, remain the gold standard, but new systems are being explored to overcome their limitations. For example, plant-based expression systems offer a cost-effective and scalable alternative, with the added benefit of reduced risk of contamination by human pathogens.
Cell-free expression systems are also gaining attention. These systems use extracts from cells to produce proteins in vitro, allowing for rapid and flexible production of antibodies. Cell-free systems can be easily scaled and adapted, providing a valuable tool for high-throughput screening.
Antibody production at ProteoGenix
At ProteoGenix, we are committed to supporting you through every stage of your custom antibody project, from generation to large-scale production. With our unique approach that combines advanced wet lab techniques with cutting-edge AI platforms, we guide you through the entire antibody production process. We provide a comprehensive suite of services, including developability assessment and improvement, selection of the best expression system for your needs, and optimization of expression conditions.
Our diverse solutions are designed to tackle your specific production challenges, ensuring success at every step. We offer a range of tools to achieve your goals, such as our market-leading XtenCHOTM proprietary mammalian cell line, our high throughput antibody production platform MultimAbXpressTM and AI-driven platforms. When you partner with ProteoGenix, you gain access to innovative and tailored solutions that meet all your development and production needs, ensuring you achieve your objectives efficiently and effectively.
Antibody production FAQ
What stimulates antibody production?
Antibody production is primarily stimulated by the presence of antigens, which are foreign substances such as pathogens (bacteria, viruses, fungi), toxins, or other foreign molecules.
How are antibodies produced?
Antibodies are produced by B cells in the immune system through a process called antibody synthesis. When B cells encounter an antigen, they are activated either directly or with the help of helper T cells. Upon activation, B cells proliferate and differentiate into plasma cells. These plasma cells undergo antibody synthesis, where they produce and secrete large quantities of antibodies specific to the encountered antigen. This synthesis process involves the transcription and translation of immunoglobulin genes within the plasma cells, ensuring the production of antibodies that can effectively target and neutralize the antigen.
What are the different steps of an antibody production process?
The production of antibodies involves several key steps. First, the antigen is prepared, often mixed with an adjuvant to enhance the immune response. Next, the selected host animal is immunized through a series of injections to stimulate the production of antibodies. For polyclonal antibodies, blood is collected from the immunized animal, and the serum, which contains the antibodies, is separated. For monoclonal antibodies, the spleen cells from the immunized animal are harvested and fused with myeloma cells to create hybridoma cells. These hybridomas are then screened for the production of the desired antibody.
Once identified, the hybridoma cells are cloned and expanded in culture to produce large quantities of the monoclonal antibody. Both polyclonal and monoclonal antibodies are then purified using techniques such as affinity chromatography. The antibodies are characterized and validated for specificity, affinity, and functional activity.
For large-scale production, the process is optimized and scaled up, ensuring consistency and quality.