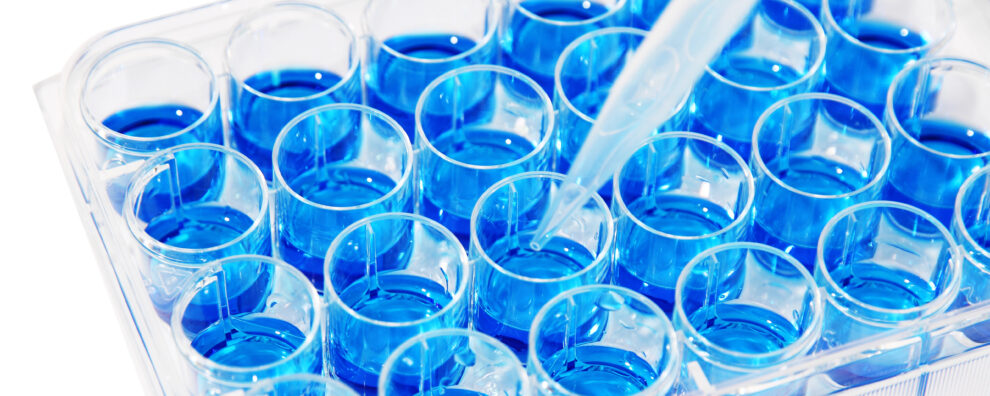
How To Improve The Yield Of Your Antibody Production?
Antibodies, or immunoglobulins, are specialized proteins produced by the immune system to identify and neutralize foreign substances such as bacteria, viruses, and other pathogens. Antibody production is a complex biological process involving the activation of B cells, which then differentiate into plasma cells capable of secreting large quantities of antibodies. In the context of the biotechnology and pharmaceutical industries, antibody production has taken a more technical approach, using recombinant DNA technology to produce monoclonal and polyclonal antibodies in various expression systems. These antibodies are essential for a wide range of applications, including therapeutic treatments, diagnostic tools, and research reagents. For scientists and researchers in the biotechnology and pharmaceutical industries, achieving high yields in antibody production is paramount. The efficiency of producing large quantities of high-quality antibodies has a direct impact on the feasibility and success of projects. High yields allow for the production of enough material for extensive testing and validation, which is critical for the development of therapeutic antibodies and diagnostic tools. In addition, the economic impact is significant; by reducing production costs, more resources can be devoted to innovative research and development. In addition, the scalability of the production processes ensures that the quality and consistency of the antibodies is not compromised when we move from the laboratory to industrial-scale production. This consistency is critical to meeting regulatory standards and ensuring the efficacy and safety of antibody-based products. In an industry where time and precision are of the essence, optimizing antibody yield is not just a goal, but a necessity that drives scientific progress and commercial success. In this article, we will explore practical strategies for improving antibody production yield, drawing on the latest research and expert insights. These strategies are designed to help you navigate and overcome common challenges, ultimately optimizing your projects and increasing the efficiency and success rates of your antibody production efforts.
- Understanding Antibody Production
- Definition and Significance of Antibodies
- Overview of Antibody Production Processes
- In Vivo vs. Recombinant Antibody Production
- Expression Systems Used in Antibody Production
- Bacterial Expression Systems
- Escherichia coli (E. coli)
- Bacillus subtilis (B.subtilis)
- Yeast Expression Systems
- Pichia pastoris
- Saccharomyces cerevisiae
- Insect Expression Systems
- Mammalian Expression Systems
- Chinese Hamster Ovary (CHO) Cells
- XtenCHO Expression System
- Human Embryonic Kidney (HEK) 293 Cells
- Other Mammalian Systems
- Factors Affecting Antibody Yield
- Gene Sequence Optimization
- Codon Optimization:
- Avoidance of Rare Codons:
- Elimination of Secondary Structures:
- Insertion of Stabilizing Elements:
- Removal of Unfavorable Sequence Motifs:
- GC Content Optimization:
- De Novo Gene Synthesis
- DNA Integration
- Stable vs. Transient Expression:
- Integration Sites:
- Expression Vectors
- Promoter Strength
- Types of Promoters
- Optimization Strategies
- Selection Markers
- Plasmid Backbone
- Enhancers and UTRs (Untranslated Regions)
- Culture Conditions
- Key Parameters and Optimization Strategies
- ProteoGenix’s Expertise in Antibody Production
- Our Services and Solutions
- Custom Cell Line Development
- Antibody Developability Assessment:
- VIPS Technology:
- XtenCHO Expression System:
- Protein Expression System Selection
- Custom Solutions:
- High-Throughput Protein Expression Screening
- Comprehensive Testing:
- Advanced Platform:
- Why Choose ProteoGenix for Antibody Production
- Comprehensive Solutions
- References
Understanding Antibody Production
Definition and Significance of Antibodies
Antibodies are critical components of the immune system, functioning as the body’s natural defense mechanism against pathogens. In the realm of biotechnology and pharmaceuticals, antibodies have transcended their biological role, becoming indispensable tools in global healthcare, diagnostics, and scientific research. The ability to produce high-yield, high-quality antibodies is vital for advancing scientific research and developing effective therapies, highlighting the importance of optimizing antibody production processes.
Overview of Antibody Production Processes
Antibody production encompasses a variety of methods, primarily divided into in vivo (animal-based) and recombinant approaches. Understanding these processes is key to improving yields and ensuring the consistent quality of antibodies.
In Vivo vs. Recombinant Antibody Production
- In Vivo Antibody Production: This traditional method involves the immunization of animals (e.g., mice, rabbits) to produce polyclonal antibodies or the use of hybridoma technology to generate monoclonal antibodies. While effective, this method is often limited by scale and variability.
- Recombinant Antibody Production: Utilizes genetic engineering to produce antibodies in various expression systems. This method offers greater control over antibody characteristics and scalability, making it the preferred choice for large-scale production.
Expression Systems Used in Antibody Production
Understanding the various expression systems available for antibody production is crucial for optimizing yield, functionality, and scalability. Each system has unique characteristics that make it suitable for different applications. Here, we provide a comprehensive overview of bacterial, yeast, mammalian, insect, and plant expression systems used in antibody production.
Bacterial Expression Systems
Escherichia coli (E. coli)
The most widely used bacterial host for recombinant protein production, including antibody fragments such as Fab and single-chain variable fragments (scFv). Its popularity stems from several key advantages:
- Rapid Growth and High Yield: E. coli can double its population approximately every 20 minutes under optimal conditions, facilitating quick production cycles. This rapid growth translates to high yield in a short period, making E. coli a cost-effective choice for large-scale production.
- Ease of Genetic Manipulation: The genetic simplicity of E. coli, along with the availability of numerous well-characterized expression vectors and promoters, allows for straightforward and efficient genetic manipulation. Techniques such as plasmid transformation and the use of strong promoters (e.g., T7 promoter) enhance protein expression levels.
- Cost-Effectiveness: E. coli culture media and fermentation processes are relatively inexpensive. The bacteria can be cultured in simple, defined media, reducing overall production costs. This makes E. coli particularly attractive for research and industrial applications where budget constraints are a concern.
- Versatility: E. coli is capable of expressing a wide range of recombinant proteins, including non-glycosylated antibody fragments. Its versatility is further enhanced by the development of various engineered strains designed to improve protein solubility and yield.
Despite these advantages, E. coli also presents several limitations that must be addressed to optimize antibody production:
- Lack of Post-Translational Modifications: E. coli lacks the cellular machinery required for complex post-translational modifications, such as glycosylation, phosphorylation, and proper disulfide bond formation. These modifications are essential for the functionality and stability of full-length antibodies. As a result, E. coli is typically used to produce antibody fragments rather than full-length antibodies.
- Inclusion Bodies: High-level expression of recombinant proteins in E. coli often leads to the formation of insoluble aggregates known as inclusion bodies. These inclusion bodies contain misfolded proteins, which must be extracted and refolded in vitro, adding complexity and cost to the production process. Strategies to mitigate this issue include optimizing expression conditions, co-expressing chaperones, and using strains engineered for enhanced protein solubility.
- Endotoxin Contamination: E. coli naturally produces endotoxins (lipopolysaccharides) that can contaminate the final product. Endotoxins can trigger severe immune responses in humans, necessitating rigorous purification processes to remove these contaminants, especially for therapeutic applications. The use of endotoxin-free E. coli strains or employing advanced purification techniques, such as affinity chromatography, can help address this challenge.
- Protein Folding and Stability: The lack of a protein chaperone system in E. coli can lead to improper folding of recombinant proteins, affecting their stability and functionality. To overcome this, researchers often optimize culture conditions, such as temperature and induction timing, and utilize specific E. coli strains designed to enhance protein folding.
Bacillus subtilis (B.subtilis)
An alternative bacterial expression system is Bacillus subtilis, which offers distinct advantages over E. coli:
- Endotoxin-Free: Unlike E. coli, B. subtilis does not produce endotoxins, making it a safer choice for producing therapeutic proteins. This reduces the burden of endotoxin removal during downstream processing.
- Secretion of Proteins: B. subtilis has a natural ability to secrete recombinant proteins directly into the culture medium, simplifying the purification process. This attribute is particularly beneficial for the production of extracellular proteins.
However, B. subtilis also has its limitations:
- Extracellular Proteases: B. subtilis produces extracellular proteases that can degrade the recombinant antibodies, reducing yield and complicating the purification process. Engineering protease-deficient strains or optimizing culture conditions can help mitigate this issue.
- Genetic Manipulation: While genetic manipulation of B. subtilis is feasible, it is generally more complex and less well-characterized compared to E. coli. This can pose challenges for researchers unfamiliar with B. subtilis genetics.
Yeast Expression Systems
Yeast expression systems are a versatile and widely used platform for recombinant antibody production. They bridge the gap between bacterial and mammalian systems by offering rapid growth and the ability to perform post-translational modifications, albeit with some differences from mammalian systems. The two most commonly used yeast species for antibody production are Pichia pastoris and Saccharomyces cerevisiae.
Pichia pastoris
It is a methylotrophic yeast that has gained prominence in recombinant protein production due to several key attributes:
- High Yield and Scalability: P. pastoris is capable of producing high yields of antibodies, making it suitable for large-scale production. It can grow to very high cell densities in bioreactors, which contributes to its high productivity.
- Post-Translational Modifications: While not as comprehensive as mammalian systems, P. pastoris can perform essential post-translational modifications such as glycosylation.
- Cost-Effectiveness: The cost of cultivation and media for P. pastoris is relatively low, and the system is less complex than mammalian cell culture. This makes it an attractive option for both research and industrial applications.
- Ease of Genetic Manipulation: P. pastoris offers a range of genetic tools and vectors that facilitate efficient genetic manipulation. Its strong promoters, such as the alcohol oxidase (AOX1) promoter, enable high-level expression of recombinant proteins.
However, P. pastoris also presents some limitations:
- Glycosylation Differences: The glycosylation patterns in P. pastoris are more similar to mammalian systems compared to other yeasts, though differences still exist and can impact the functionality and immunogenicity of antibodies.
- Methanol Induction: The use of methanol as an inducer for protein expression under the AOX1 promoter requires careful handling and can be a safety concern at large scales.
Saccharomyces cerevisiae
There are commonly known as baker’s yeast, is another widely used yeast expression system. It offers several advantages:
- Well-Characterized System: S. cerevisiae has a long history of use in biotechnology, and its genetics are well understood. This extensive knowledge base facilitates genetic manipulation and optimization for protein production.
- Rapid Growth and Simple Cultivation: S. cerevisiae grows rapidly and can be cultured in simple, inexpensive media. This makes it cost-effective and easy to scale up for large production runs.
- Post-Translational Modifications: S. cerevisiae can perform some post-translational modifications, such as glycosylation and phosphorylation, though these modifications differ from those in mammalian cells.
Despite these benefits, S. cerevisiae has notable limitations:
- Hyper-Glycosylation: One of the main challenges with S. cerevisiae is its tendency to hyper-glycosylate proteins, adding excessive mannose residues. This hyper-glycosylation can affect the stability, solubility, and immunogenicity of antibodies.
- Secretion Efficiency: While S. cerevisiae can secrete proteins, the efficiency of secretion can vary, and optimizing secretion signals is necessary to achieve high yields of functional antibodies.
Insect Expression Systems
Insect expression systems, particularly those utilizing the Baculovirus Expression Vector System (BEVS), are valuable tools for recombinant antibody production. These systems combine the advantages of eukaryotic post-translational modifications with relatively high yield and scalability. This section provides a comprehensive overview of insect expression systems, focusing on their mechanisms, benefits, and limitations in antibody production.
- Advantages of Insect Expression Systems
- High Expression Levels: The baculovirus system can achieve high expression levels, producing substantial amounts of recombinant proteins in a relatively short time frame. This is particularly useful for producing proteins that are difficult to express in other systems.
- Post-Translational Modifications: Insect cells can perform essential post-translational modifications, enhancing the functionality and stability of the produced antibodies. While not identical to mammalian modifications, these modifications are often sufficient for many research and industrial applications.
- Safety: Baculoviruses are not pathogenic to humans, making the system safe to work with in laboratory and industrial settings. Additionally, insect cells are less susceptible to contamination with human pathogens, reducing the risk of viral contamination in the final product.
- Limitations of Insect Expression Systems
- Glycosylation Differences: The glycosylation patterns in insect cells differ from those in mammalian systems. Insect cells typically add high-mannose glycans, which can affect the therapeutic efficacy and immunogenicity of the antibodies. Efforts are ongoing to engineer insect cell lines with more human-like glycosylation patterns.
- Batch-to-Batch Variability: The production process in insect systems is typically batch-based, which can lead to variability between batches. This variability can affect the consistency and quality of the produced antibodies, posing challenges for large-scale production.
- Cell Lysis: The baculovirus infection process eventually leads to cell lysis, which releases the recombinant protein into the culture medium. This necessitates additional purification steps to separate the protein from cellular debris and other contaminants.
- Complex Culture Conditions: While insect cells are more robust than mammalian cells, they still require specific culture conditions, including precise temperature and nutrient control. Optimizing these conditions is essential to maximize yield and maintain cell health.
Mammalian Expression Systems
Mammalian expression systems are the gold standard for antibody production, especially for therapeutic applications. These systems offer several advantages, including the ability to perform complex post-translational modifications and produce proteins that closely mimic their natural counterparts.
Chinese Hamster Ovary (CHO) Cells
The most widely used mammalian cell line for the production of therapeutic antibodies. They have been extensively characterized and optimized for high-level protein expression, making them the preferred choice for large-scale production. CHO cells are transfected with plasmids containing the genes encoding the antibody’s heavy and light chains. These cells then use their cellular machinery to produce and secrete the recombinant antibody. CHO cells can be cultured in suspension, allowing for scalable production in bioreactors.
- Advantages of CHO Cells
- Post-Translational Modifications: One of the key advantages of CHO cells is their ability to perform human-like post-translational modifications, including glycosylation, phosphorylation, and proper disulfide bond formation. These modifications are crucial for the stability, functionality, and therapeutic efficacy of antibodies.
- High Yield and Scalability: CHO cells can achieve high expression levels, often producing grams of antibody per liter of culture. They can be adapted to serum-free, chemically defined media, which improves reproducibility and reduces the risk of contamination. This scalability makes CHO cells suitable for both clinical and commercial production.
- Regulatory Acceptance: CHO cells are well-accepted by regulatory agencies worldwide, which facilitates the approval process for biopharmaceuticals produced using this system.
- Genetic Stability: CHO cells exhibit relatively stable expression of recombinant proteins over multiple generations, which is important for maintaining consistent production levels.
- Limitations of CHO Cells
- High Cost: The cost of media and the need for sophisticated bioreactors make CHO cell cultures more expensive than bacterial or yeast systems.
- Long Development Time: The process of developing stable CHO cell lines and optimizing production conditions can be time-consuming, often taking several months to years.
XtenCHO Expression System
The XtenCHO expression system is a state-of-the-art platform designed to maximize the efficiency and yield of antibody production in CHO cells. This system integrates several optimized components to address the traditional limitations of CHO cell expression:
- Enhanced Transfection Efficiency: The XtenCHO system uses advanced transfection reagents and protocols that significantly enhance the efficiency of gene delivery into CHO cells. This improvement in transfection efficiency results in higher initial expression levels of the recombinant protein, facilitating more robust production outcomes.
- High Yield: The combination of optimized transfection, growth media, and stable cell line development results in significantly higher yields of recombinant proteins compared to traditional CHO cell systems.
- Optimized Growth Media: The XtenCHO system utilizes a specifically formulated growth medium that promotes higher cell densities and improves overall cell health. This medium is tailored to work synergistically with the XtenCHO cells, thereby maximizing antibody yield.
- Stable Cell Line Development: XtenCHO offers streamlined protocols for developing stable CHO cell lines, reducing the time required to achieve high-producing clones with consistent and reproducible results. This stability is crucial for large-scale production and regulatory compliance.
- Scalability: This system is scalable from small laboratory cultures to large industrial bioreactors, making it suitable for both research and commercial production.
Human Embryonic Kidney (HEK) 293 Cells
HEK 293 cells are another popular mammalian expression system known for their high transfection efficiency and ease of use. They are transfected with plasmids carrying the antibody genes. These cells are particularly suited for transient transfection, which allows for rapid production of antibodies without the need for stable cell line development.
- Post-Translational Modifications: HEK 293 cells perform human-like post-translational modifications, including glycosylation, ensuring that the produced antibodies are functionally similar to those produced in humans.
- Ease of Use and Rapid Production: HEK 293 cells are known for their high transfection efficiency, which facilitates rapid production of recombinant proteins. This makes them ideal for producing small to medium quantities of antibodies for research and preclinical studies.
- Limitations of HEK 293 Cells
- Susceptibility to Human Viruses: HEK 293 cells are derived from human tissue and are more susceptible to human viruses, which can pose a contamination risk.
- Lower Yield: Compared to CHO cells, HEK 293 cells generally produce lower yields of recombinant proteins, making them less suitable for large-scale production.
Other Mammalian Systems
In addition to CHO and HEK 293 cells, other mammalian cell lines such as NS0 (murine myeloma cells) and BHK (Baby Hamster Kidney) cells are also used for antibody production. These systems offer unique advantages but are less commonly used due to specific limitations related to yield, scalability, and regulatory acceptance.
Comparison Table of Expression Systems
Expression System | Advantages | Limitations |
---|---|---|
E. coli | Rapid growth, cost-effective, easy genetic manipulation | Lacks complex PTMs, inclusion bodies, endotoxin contamination |
B. subtilis | Endotoxin-free, secretes proteins | Produces extracellular proteases, more complex genetic manipulation |
Pichia pastoris | High yield, cost-effective, performs essential PTMs | Glycosylation differences, methanol induction required |
S. cerevisiae | Well-characterized, rapid growth, simple cultivation | Hyper-glycosylation, variable secretion efficiency |
CHO Cells | Human-like PTMs, scalable, high yield | High cost, longer development times, genetic instability |
HEK 293 Cells | Human-compatible glycosylation, high transfection efficiency | Susceptible to human viruses |
Insect Cells (BEVS) | High expression levels, performs some PTMs | Glycosylation differences, batch inconsistencies |
Factors Affecting Antibody Yield
Gene Sequence Optimization
Gene sequence optimization is the process of altering the nucleotide sequence of a gene to enhance its expression and functionality in a specific host organism. This optimization is crucial for improving the yield, stability, and activity of recombinant proteins, including antibodies. The primary goal is to tailor the gene sequence to be more efficiently transcribed and translated by the host cell’s machinery, thereby increasing the overall production of the desired protein. This is particularly important because antibodies are complex proteins that require proper folding, post-translational modifications, and assembly.
Codon Optimization:
Codon optimization is the practice of modifying the codon sequence of a gene to match the codon usage preferences of the host organism. This is crucial because different organisms exhibit preferences for certain codons over others, known as codon bias. By adjusting the codon usage in the gene sequence to match the preferred codons of the host organism, the translation process can be made more efficient. This helps to avoid issues such as ribosomal stalling and can significantly increase protein yield. For example, while the codon usage in E. coli is different from that in mammalian cells, optimizing the codons for the specific host can lead to improved expression levels.
Avoidance of Rare Codons:
In some hosts, certain codons are rare and correspond to tRNAs that are in low abundance. Using these rare codons can slow down translation and reduce protein yield. Gene sequence optimization replaces these rare codons with more common ones that are readily available in the host, ensuring a smoother and faster translation process.
Elimination of Secondary Structures:
DNA and mRNA secondary structures, such as hairpins, can impede the transcription and translation processes. By redesigning the gene sequence to minimize these structures, the efficiency of gene expression can be enhanced. Secondary structures in mRNA can cause ribosomes to stall or fall off the mRNA, reducing the efficiency of translation and thus the yield of the protein.
Insertion of Stabilizing Elements:
Incorporating elements such as Kozak sequences (for eukaryotic systems) or Shine-Dalgarno sequences (for prokaryotic systems) near the start codon can enhance the initiation of translation. These sequences help ribosomes to recognize the start site more effectively, increasing the translation efficiency of the mRNA.
Removal of Unfavorable Sequence Motifs:
Certain sequences, such as those that create premature termination signals or splice sites, can negatively impact gene expression. Identifying and modifying these sequences can prevent unintended interruptions in the transcription and translation processes. For example, internal ribosome entry sites (IRES) and cryptic splice sites can be modified or removed to improve mRNA stability and translation efficiency.
GC Content Optimization:
The GC content of a gene can affect its stability and the efficiency of its expression. Genes with extremely high or low GC content can be difficult to transcribe efficiently. Balancing the GC content to an optimal level for the host can enhance expression. High GC content can lead to stable mRNA structures that are resistant to degradation, but extremely high GC content can also make transcription initiation more difficult. An optimal balance is crucial for efficient gene expression.
De Novo Gene Synthesis
Modern gene synthesis technologies allow for the precise construction of optimized gene sequences from scratch. This means that researchers can design genes without the constraints of naturally occurring sequences, incorporating all necessary optimizations from the start. De novo synthesis allows for the inclusion of additional features such as purification tags or regulatory elements, further enhancing the utility of the synthesized gene. This process involves:
- Design: Using bioinformatics tools to design the optimized gene sequence
- Synthesis of Optimized Genes: Advances in synthetic biology have made it possible to design and synthesize genes with optimized codon usage and other beneficial modifications. This allows for the creation of completely synthetic genes that are tailored for high expression in specific host systems.
- Assembly: Combine shorter oligonucleotides into longer gene sequences using techniques like Polymerase Chain Reaction (PCR) or Ligase Chain Reaction (LCR).
- Cloning and Verification: Clone the synthesized gene into an appropriate vector and verify its sequence accuracy through sequencing.
DNA Integration
DNA integration refers to the stable incorporation of foreign DNA into the host cell’s genome. This process is crucial for long-term expression of the recombinant gene, particularly in large-scale and therapeutic antibody production where consistent and stable gene expression is required. Proper DNA integration significantly improves antibody yield by ensuring that the gene of interest is consistently expressed over multiple generations of cells.
Stable vs. Transient Expression:
In transient expression, the introduced DNA is not integrated into the host genome and is eventually lost as the cells divide. This method is useful for short-term studies and rapid protein production, but it is not suitable for long-term production due to the decline in gene expression over time. Stable expression, on the other hand, involves integrating the DNA into the host genome, ensuring that the gene is maintained and expressed in all daughter cells over many generations. This stability is essential for continuous and reliable antibody production, leading to higher overall yields.
Integration Sites:
The site of DNA integration can affect the level of gene expression. Random integration can lead to position effects where the surrounding chromatin structure influences gene expression. Targeted integration into “safe harbor” sites in the genome, which are known to support high and consistent expression, can mitigate these effects. Proper site selection for integration ensures that the antibody genes are expressed at optimal levels, enhancing yield.
Expression Vectors
Expression vectors are essential tools in the production of recombinant antibodies, as they significantly influence the expression levels, stability, and yield of the desired antibodies. These vectors are engineered plasmids or viruses designed to carry the gene of interest into the host cells. By incorporating specific elements that enhance transcription and translation, these vectors facilitate efficient protein production, ultimately improving antibody yield. Here, we delve into the critical components and considerations in optimizing expression vectors to maximize antibody yield.
Promoter Strength
They are DNA sequences that control the initiation of transcription. The strength of a promoter directly affects the rate at which the gene is transcribed into mRNA, with strong promoters leading to high levels of mRNA and significantly enhancing antibody production. Commonly used strong promoters include the cytomegalovirus (CMV) promoter in mammalian systems and the T7 promoter in bacterial systems. By choosing a promoter that is highly compatible with the host cell’s transcription machinery, researchers can maximize gene expression, thereby increasing the overall yield of antibodies.
Types of Promoters
- Constitutive Promoters: These promoters are active continuously, leading to constant gene expression. Examples include the CMV (cytomegalovirus) promoter used in mammalian systems and the T7 promoter used in bacterial systems. Constitutive promoters are useful for applications requiring steady-state expression levels.
- Inducible Promoters: Inducible promoters can be turned on or off in response to specific stimuli, such as chemical inducers or environmental conditions. Examples include the lac promoter in E. coli, which is induced by IPTG, and the AOX1 promoter in Pichia pastoris, which is induced by methanol. Inducible promoters are advantageous for controlling the timing and level of gene expression, reducing potential toxicity and metabolic burden on the host cells.
Optimization Strategies
- Promoter Selection: Choosing the right promoter based on the host cell type and the desired expression profile is crucial. For example, using the CMV promoter in mammalian cells or the T7 promoter in bacterial systems ensures compatibility and high expression levels.
- Promoter Engineering: Modifying promoter sequences to enhance their strength can further boost transcriptional activity. Techniques such as site-directed mutagenesis or the inclusion of enhancer elements can increase promoter efficiency.
- Contextual Factors: The position and context of the promoter within the vector can influence its activity. Ensuring that the promoter is placed in an optimal location, free from inhibitory elements, can enhance its effectiveness.
- Synthetic Promoters: Designing synthetic promoters that combine elements from different natural promoters can create customized sequences with desired strengths and regulatory properties. These synthetic promoters can be tailored to specific production needs.
Selection Markers
Selection markers are genes included in the expression vector that confer resistance to specific antibiotics or other selective agents, allowing for the identification and maintenance of cells that have successfully taken up the expression vector. Common selection markers include genes for antibiotic resistance, such as ampicillin, kanamycin, and hygromycin. Applying selective pressure ensures that only cells harboring the expression vector survive, creating a homogeneous population of high-producing cells. This homogeneous cell population is crucial for achieving consistent and high yields of antibodies.
Plasmid Backbone
The plasmid backbone includes structural elements of the vector such as the origin of replication, multiple cloning sites, and selection markers. The origin of replication controls the number of plasmid copies within the cell, influencing the gene dosage and thus the level of protein production. High-copy plasmids can lead to greater yields but may also impose a metabolic burden on the host cell. Balancing plasmid copy number is essential for maintaining cell health and optimizing production, which ultimately improves antibody yield.
Enhancers and UTRs (Untranslated Regions)
Enhancers are regulatory DNA sequences that can increase the transcriptional activity of promoters. When included in the expression vector, enhancers can boost overall expression levels of the gene of interest. Similarly, untranslated regions (UTRs) flanking the coding sequence can influence mRNA stability, translation efficiency, and localization. Optimizing these regions can lead to higher and more stable expression of the recombinant antibody, thereby improving yield.
Culture Conditions
Optimizing culture conditions is a fundamental aspect of improving antibody yield in recombinant production systems. The environment in which host cells are cultured significantly affects their growth, productivity, and the quality of the antibodies produced. Understanding and fine-tuning these conditions can lead to substantial increases in antibody yield, making the production process more efficient and cost-effective. This section provides a comprehensive overview of the key parameters and strategies involved in optimizing culture conditions for antibody production.
Key Parameters and Optimization Strategies
- Temperature: is a crucial factor in cell culture, affecting cellular metabolism, protein folding, and stability. Most mammalian cells, such as Chinese Hamster Ovary (CHO) cells, are typically cultured at 37°C. However, studies have shown that reducing the temperature slightly during the production phase (to 30-33°C) can enhance the quality and yield of antibodies. Lower temperatures slow down the cellular metabolism, allowing more time for proper protein folding and reducing the formation of misfolded proteins and aggregates.
Implementing a temperature shift strategy by initially culturing cells at a higher temperature (e.g., 37°C) for rapid growth, followed by a reduction to 30-33°C during the production phase to improve protein folding and stability. - Maintaining an optimal pH is essential for cell viability and productivity. The optimal pH range for mammalian cell cultures is generally around 7.2-7.4. Deviations from this range can negatively impact enzyme activity and nutrient availability, ultimately affecting cell growth and antibody production.
Using automated pH control systems to monitor and adjust pH continuously, as well as buffering agents and controlled addition of acids or bases can help maintain a stable environment. - Dissolved Oxygen (DO) constitutes a vital element for cellular respiration and energy production. The optimal DO levels for mammalian cells are typically maintained at 30-40% of air saturation. Both low and high oxygen levels can have detrimental effects: low levels can limit cell growth and productivity, while high levels can be toxic.
- Nutrient availability is a key determinant of cell growth and productivity. The culture media must provide all essential nutrients, including amino acids, vitamins, minerals, and energy sources such as glucose. Optimized feeding strategies, such as fed-batch and perfusion methods, can continuously supply nutrients to prevent depletion and support high-density cell cultures.
ProteoGenix’s Expertise in Antibody Production
ProteoGenix is at the forefront of antibody production, offering a range of services designed to maximize antibody production yield and ensure the highest quality of antibodies. Our expertise spans custom cell line development, protein expression system selection, and high-throughput protein expression screening. Here’s an in-depth look at our services and why you should choose ProteoGenix for your antibody production needs.
Our Services and Solutions
Custom Cell Line Development
The development of stable cell lines is a critical step in the clinical development of monoclonal antibodies. Our approach involves extensive early testing of antibody leads to ensure they meet the best stability, reactivity, and effectiveness before committing to large-scale production. This process includes:
Antibody Developability Assessment:
At ProteoGenix, we prioritize early developability assessment to ensure antibodies meet the highest standards of stability, reactivity, and effectiveness. This involves analyzing key characteristics such as aggregation, affinity, specificity, and glycosylation profiles through in silico methods and recombinant antibody production in our facilities. By addressing these factors early, we can bypass many stable expression hurdles and ensure optimal production.
VIPS Technology:
The Verified In-Situ Plate Screening (VIPS) technology at ProteoGenix enables rapid screening of clones, ensuring the selection of the best, high-producing cell lines. This technology provides a double lock of clonality assurance for regulatory compliance and provides automated documentation for traceability for IND submissions.
XtenCHO Expression System:
Our proprietary XtenCHO expression system is engineered for superior antibody production. It combines enhanced transfection efficiency, optimized growth media, and stable cell line development to achieve significantly higher yields compared to traditional CHO cell systems. The XtenCHO system is scalable from laboratory to industrial bioreactors, making it ideal for both research and commercial production.
Learn more about our Custom Cell Line Development services
Protein Expression System Selection
Selecting the appropriate antibody expression system is crucial for achieving optimal activity and yield. ProteoGenix offers a multi-system expression service, allowing us to tailor solutions to your specific needs:
Custom Solutions:
We can utilize 2 to 5 different expression systems to find the best match for your project requirements, ensuring high productivity and functional activity.
Explore our protein expression system selection services
High-Throughput Protein Expression Screening
For challenging expression projects, our high-throughput screening platform is designed to test a wide range of conditions rapidly:
Comprehensive Testing:
Up to 1000 conditions in 4 weeks, focusing on productivity, purity, solubility, stability, and biological activity.
Advanced Platform:
Our platform leverages the expertise of top protein production scientists to optimize expression conditions, ensuring successful antibody production.
Discover our high-throughput protein expression screening services
Why Choose ProteoGenix for Antibody Production
Comprehensive Solutions
Beyond our specialized services, ProteoGenix offers a range of additional advantages:
- Expertise Across Expression Systems: With over 28 years of experience in protein production using mammalian, bacterial, yeast, and insect systems, we can handle diverse and complex projects.
- Regulatory Compliance: Our processes are designed to meet stringent regulatory standards, ensuring that our products are safe and effective for therapeutic use.
- Customer-Centric Approach: Our PhD account managers work closely with clients to understand their needs and provide tailored solutions, ensuring the best possible outcomes for every project.
Choosing ProteoGenix means leveraging our extensive expertise, innovative technologies, and commitment to quality, all of which contribute to higher antibody yields and successful project outcomes. Book a call with our experts today to discuss your project needs!
References
Verma, R., Boleti, E., & George, A. J. T. (1998). Antibody engineering: comparison of bacterial, yeast, insect and mammalian expression systems. Journal of Immunological Methods, 216(1-2), 165-181. DOI: 10.1016/S0022-1759(98)00077-5
Ahmadi, M., et al. (2017). PhiC31 integrase can improve the efficiency of different construct designs for monoclonal antibody expression in CHO cells. Protein Expression and Purification, 134, 89-95. DOI: 10.1016/j.pep.2017.04.005