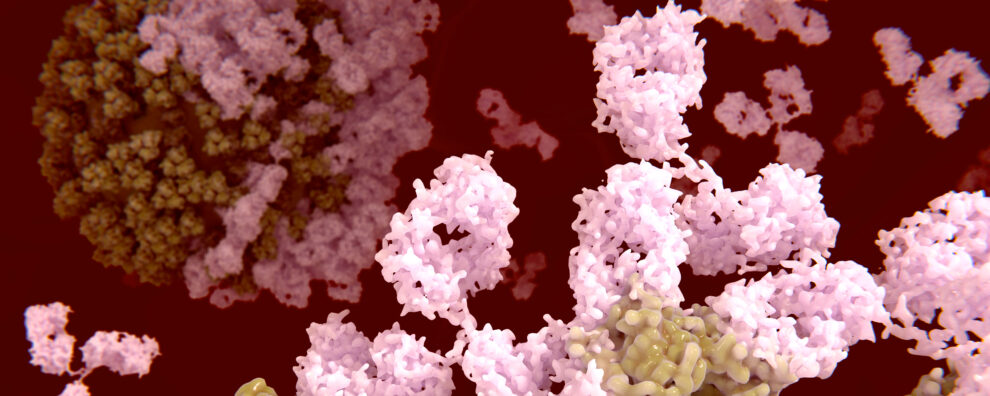
Cracking the Code: How to Achieve the Perfect Balance of Affinity and Specificity in Antibodies
The development of monoclonal antibodies (mAbs) has revolutionized therapeutic and diagnostic applications, offering unparalleled precision in targeting diseases. Achieving the perfect balance of high affinity and specificity in these antibodies is crucial for their success. High affinity ensures robust binding to the target antigen, essential for therapeutic efficacy, while specificity guarantees that the antibody targets only the intended antigen, minimizing off-target effects and potential toxicity. However, optimizing these two parameters simultaneously is a complex challenge that often involves trade-offs. Researchers have developed innovative strategies to overcome these challenges, such as high-throughput screening techniques like phage and yeast display, and advanced computer-aided design methods. Affinity maturation through targeted mutations further refines antibody properties, ensuring both strong binding and precise targeting. This blog is your comprehensive guide to cracking the code of antibody development. We aim to provide an in-depth exploration of how to achieve the perfect balance of affinity and specificity in antibodies. You'll discover the latest advancements and cutting-edge methodologies in antibody engineering, including the use of phage display, yeast display, and computer-aided design. We will also delve into the challenges researchers face in optimizing these critical parameters and propose practical solutions based on recent scientific literature. By the end of this blog, you'll have a clear understanding of the strategies available to develop high-affinity, high-specificity antibodies for various applications in therapeutics, diagnostics, and research. Dive in to revolutionize your research and applications with these innovative strategies.
- Understanding Affinity and Specificity in Antibodies
- Definitions and Importance
- Historical Development
- Current Trends and Innovations
- The Significance of High Affinity in Antibodies
- Mechanisms of Antibody Affinity
- Techniques for Measuring Affinity
- The Role of Specificity in Antibody Function
- Defining Specificity and Its Implications
- Assessing Specificity
- Applications of High-Affinity and High-Specificity Antibodies
- Balancing Affinity and Specificity: Challenges and Solutions
- Common Challenges in Antibody Development
- Strategies for Achieving Optimal Balance
- Techniques for Enhancing Antibody Affinity and Specificity
- Phage Display and Other Library Technologies
- Computational Approaches and In Silico Modeling
- Site-Directed Mutagenesis and Affinity Maturation
- Techniques for Enhancing Antibody Affinity and Specificity
- Phage Display and Other Library Technologies
- Computational Approaches and In Silico Modeling
- Site-Directed Mutagenesis and Affinity Maturation
- Future Directions in Antibody Development
- Emerging Technologies and Techniques
- ProteoGenix’s Services for Optimal Antibody Development
- Overview of ProteoGenix’s Expertise and Capabilities
- Affinity Maturation via AI
- Benefits and Applications of Phage Display at ProteoGenix
- Conclusion
- Resources
Understanding Affinity and Specificity in Antibodies
Definitions and Importance
- Affinity
Affinity refers to the strength of the interaction between an antibody and its specific antigen. This interaction is driven by non-covalent forces such as hydrogen bonds, van der Waals forces, electrostatic interactions, and hydrophobic effects. High-affinity antibodies bind more tightly to their antigens, ensuring robust and stable interactions. This characteristic is crucial for therapeutic antibodies, as it determines the efficacy of the antibody in neutralizing pathogens or targeting diseased cells. In diagnostics, high affinity enhances the sensitivity and reliability of detection assays, allowing for the identification of low-abundance targets.
- Specificity
Specificity is the ability of an antibody to distinguish between different antigens and bind exclusively to its target antigen. High specificity minimizes off-target interactions, which is essential for reducing potential side effects in therapeutic applications and preventing false positives in diagnostic tests. Specificity is determined by the unique structure of the antibody’s binding site, which is shaped to fit a particular antigen. This precision ensures that antibodies bind only to the intended targets, making them powerful tools in both clinical and research settings.
Historical Development
The field of antibody engineering has seen remarkable advancements since the inception of monoclonal antibody technology. The landmark development was the creation of hybridoma technology by Köhler and Milstein in 1975, which enabled the production of monoclonal antibodies. This technique involves fusing myeloma cells with spleen cells from an immunized mouse, resulting in hybrid cells that can produce identical antibodies indefinitely. This breakthrough earned them the Nobel Prize in Physiology or Medicine in 1984.
In the subsequent decades, recombinant DNA technology revolutionized antibody engineering. The introduction of phage display technology in the late 1980s and early 1990s marked a significant milestone. Phage display allows for the expression of antibody fragments on the surface of bacteriophages, facilitating the screening of large libraries to identify antibodies with desired properties. This method, pioneered by George P. Smith and Sir Gregory P. Winter, significantly accelerated the development of antibodies with enhanced affinity and specificity.
Another major advancement was the development of humanized and fully human antibodies. Initially, most therapeutic antibodies were derived from mouse models, which posed immunogenicity issues when used in humans. Techniques such as CDR grafting and transgenic mice capable of producing human antibodies have mitigated these problems, leading to the creation of antibodies that are less likely to elicit immune responses in patients.
Current Trends and Innovations
- Phage Display and Yeast Display
Phage display remains a cornerstone of antibody engineering, allowing researchers to screen vast libraries of antibody variants to identify those with optimal affinity and specificity. Yeast display is another powerful technology where antibody fragments are expressed on the surface of yeast cells, enabling the selection of antibodies with improved binding characteristics. These display technologies facilitate the high-throughput screening and rapid optimization of antibodies, making them indispensable tools in modern antibody development.
- Computer-Aided Design (In Silico Modeling)
Computational tools have become integral to antibody engineering. In silico modeling and molecular docking techniques allow researchers to predict how specific mutations will affect the structure and binding properties of antibodies. These tools enable the rational design of antibodies with enhanced affinity and specificity, reducing the need for extensive experimental screening. Software such as BioLuminate and MOE are commonly used for these purposes, providing detailed insights into antibody-antigen interactions at the atomic level.
- Affinity Maturation
Affinity maturation is a process that involves introducing mutations into the antibody’s variable regions to enhance binding strength. Techniques such as error-prone PCR, DNA shuffling, and site-directed mutagenesis are used to create libraries of mutated antibodies. These libraries are then screened to identify variants with improved affinity. High-throughput screening methods and computational tools have significantly streamlined this process, allowing for the rapid optimization of antibody candidates.
- Next-Generation Sequencing (NGS)
Next-generation sequencing technologies have revolutionized the way antibody repertoires are analyzed. NGS allows for the rapid and comprehensive sequencing of antibody libraries, providing detailed information about the diversity and evolution of antibody responses. This data is invaluable for guiding the design and optimization of therapeutic antibodies, enabling the identification of the most promising candidates with high affinity and specificity.
- Artificial Intelligence and Machine Learning
Artificial intelligence (AI) and machine learning are increasingly being applied to antibody engineering. These technologies analyze large datasets to identify patterns and predict the effects of specific mutations on antibody properties. AI-driven platforms can accelerate the development of antibodies by providing insights that would be difficult to obtain through traditional methods. This approach enhances the efficiency of antibody optimization and increases the likelihood of success in creating antibodies with optimal characteristics.
The Significance of High Affinity in Antibodies
Mechanisms of Antibody Affinity
Biochemical Interactions Involved
Antibody affinity is governed by a complex interplay of non-covalent biochemical interactions between the antibody’s paratope and the antigen’s epitope. These interactions include:
- Hydrogen Bonds: These occur between polar groups of the antibody and the antigen, contributing significantly to binding strength and specificity.
- Van der Waals Forces: These weak, short-range forces arise from transient dipoles in atoms and molecules, enhancing the overall binding stability.
- Electrostatic Interactions: Ionic bonds between charged residues of the antibody and antigen play a crucial role in the initial attraction and stabilization of the antibody-antigen complex.
- Hydrophobic Interactions: Non-polar regions of the antibody and antigen tend to avoid water and cluster together, driving the binding process in aqueous environments.
The combined effect of these interactions results in a high-affinity bond that is both strong and specific, ensuring that the antibody can effectively recognize and neutralize its target antigen.
Techniques for Measuring Affinity
- Surface Plasmon Resonance (SPR)
Surface Plasmon Resonance is a powerful technique used to measure the real-time interaction between an antibody and its antigen without labeling. It involves immobilizing one interaction partner on a sensor chip and flowing the other partner over it. The binding interactions alter the refractive index near the sensor surface, which is detected and analyzed to provide kinetic data, including the association rate (ka), dissociation rate (kd), and equilibrium dissociation constant (Kd). SPR is widely used due to its high sensitivity and ability to provide detailed kinetic profiles of antibody-antigen interactions.
- Biolayer Interferometry (BLI)
Biolayer Interferometry is another label-free technique that measures changes in the optical thickness of a biosensor surface upon binding of an antibody to its antigen. This method uses a fiber optic biosensor dipped into solutions containing the interaction partners. BLI provides real-time kinetic data similar to SPR but with a simpler setup and often lower cost. It is particularly useful for high-throughput screening and characterization of antibody interactions.
- Enzyme-Linked Immunosorbent Assay (ELISA)
ELISA is a well-established technique used to quantify the binding affinity of antibodies. It involves immobilizing the antigen on a plate, followed by incubation with the antibody. Detection is typically achieved using an enzyme-linked secondary antibody that produces a colorimetric or fluorescent signal proportional to the amount of antibody bound to the antigen. Although ELISA does not provide real-time kinetic data, it is highly sensitive and versatile, making it a staple in affinity measurement.
The Role of Specificity in Antibody Function
Defining Specificity and Its Implications
Specificity in antibodies refers to the precise ability to bind to a particular antigen while discriminating against structurally similar molecules. This selectivity is governed by the antibody’s variable region, particularly the complementarity-determining regions (CDRs), which form the antigen-binding site. Specificity is a critical attribute for antibodies, as it determines the fidelity with which they can distinguish between different antigens, a property that is especially crucial in complex biological environments.
The importance of avoiding cross-reactivity lies in minimizing unintended interactions with non-target antigens, which can lead to inaccurate results in diagnostic assays and adverse effects in therapeutic applications. Cross-reactivity can cause false positives, misleading disease diagnoses, and potential off-target toxicity when antibodies bind to unintended targets. Thus, achieving high specificity is paramount to ensuring the effectiveness and safety of antibodies in both clinical and research settings.
Assessing Specificity
- Western Blotting
Western blotting is a fundamental technique for evaluating antibody specificity. It involves the separation of proteins by gel electrophoresis, followed by their transfer onto a membrane and subsequent probing with the antibody. The presence of specific bands corresponding to the target protein indicates the antibody’s ability to specifically recognize its intended antigen. However, the detection of additional bands may suggest cross-reactivity, necessitating further validation and optimization.
- Immunohistochemistry (IHC)
Immunohistochemistry is used to assess antibody specificity within the spatial context of tissue samples. By binding antibodies to specific antigens in tissue sections, followed by visualization using enzyme-conjugated or fluorescent secondary antibodies, IHC provides insights into the localization and expression patterns of the target antigen. This technique is particularly useful for confirming the specificity of antibodies in situ, ensuring that they bind exclusively to the intended antigen within a complex tissue environment.
- Flow Cytometry
Flow cytometry is a highly sensitive technique for assessing the specificity of antibodies, particularly those targeting cell surface markers. It involves labeling cells with fluorescently tagged antibodies and passing them through a flow cytometer. The fluorescence intensity, which correlates with antibody binding, is measured for each cell. This method allows for the quantitative analysis of antigen expression on individual cells within heterogeneous populations, providing a detailed assessment of an antibody’s specificity and cross-reactivity.
Applications of High-Affinity and High-Specificity Antibodies
High-affinity and high-specificity antibodies are invaluable in both research and clinical diagnostics due to their precise binding capabilities and strong interactions with target antigens. These properties ensure accurate detection, quantification, and characterization of biomolecules, making them essential tools across various scientific and medical disciplines.
- Research
In biomedical research, high-affinity and high-specificity antibodies are critical for the precise detection and quantification of proteins. They are utilized in a variety of assays, including Western blotting, immunoprecipitation, and enzyme-linked immunosorbent assays (ELISA). These techniques rely on the antibody’s ability to specifically recognize and bind to target proteins, allowing researchers to study protein expression levels, post-translational modifications, and protein-protein interactions.
Moreover, these antibodies facilitate the isolation and characterization of cellular components, aiding in the exploration of cellular pathways, signal transduction, and the molecular mechanisms underlying diseases. The high specificity of these antibodies ensures that experimental results are accurate and free from interference by non-specific binding, thereby providing reliable data that reflect the true biological state of the samples being studied.
- Diagnostics
In clinical diagnostics, high-affinity and high-specificity antibodies are crucial for the accurate detection of disease-associated biomarkers. They are employed in a wide range of diagnostic assays, such as immunoassays, lateral flow tests, and biosensors. These assays depend on the antibodies’ ability to selectively bind to specific antigens present in patient samples, allowing for the identification and quantification of biomarkers indicative of diseases.
The specificity of these antibodies is particularly important for ensuring the accuracy of diagnostic tests. It prevents cross-reactivity with other similar molecules, which could otherwise lead to false positives or false negatives. For example, highly specific antibodies against unique viral epitopes are essential in serological tests for detecting infections like HIV or hepatitis. These tests must accurately differentiate between the presence of disease-specific antigens and other potential interfering substances in the sample, providing reliable and clinically relevant results.
Furthermore, high-affinity antibodies enhance the sensitivity of diagnostic assays, enabling the detection of low-abundance biomarkers. This sensitivity is vital for early disease diagnosis, monitoring disease progression, and assessing patient responses to treatment. The ability to detect and quantify these biomarkers with high precision makes these antibodies indispensable in both routine clinical testing and advanced medical research.
Balancing Affinity and Specificity: Challenges and Solutions
The development of monoclonal antibodies with high affinity and specificity is critical for their effectiveness in therapeutic and diagnostic applications. However, achieving a balance between these two properties poses significant challenges. Enhancing affinity can sometimes lead to increased non-specific binding, while improving specificity might compromise the binding strength. This section explores the common challenges in antibody development and the strategies employed to achieve an optimal balance.
Common Challenges in Antibody Development
Trade-offs Between Affinity and Specificity
- Trade-offs Between Affinity and Specificity
Balancing affinity and specificity in antibody engineering is a complex challenge that requires careful consideration of various factors. High-affinity antibodies are desirable because they bind tightly to their target antigens, which enhances therapeutic efficacy and diagnostic sensitivity. However, increasing affinity can sometimes reduce specificity, leading to undesirable cross-reactivity. This trade-off occurs because mutations that enhance binding strength—often through increased hydrophobic or electrostatic interactions—can also promote non-specific binding. For instance, studies have shown that an increased arginine content in the CDRs of antibodies can decrease specificity, resulting in off-target interactions.
- Chemical Diversity in CDRs
The challenge of maintaining specificity while optimizing affinity is further complicated by the vast chemical diversity within antibody CDRs. Theoretically, the sequence space is immense, making it difficult to predict which combinations of amino acids will achieve the desired balance of properties. This difficulty is especially pronounced during the affinity maturation process, where antibodies are subjected to rounds of mutation and selection to enhance their binding properties. While this process can significantly improve affinity, it can also inadvertently increase the likelihood of cross-reactivity, as mutations that improve binding to the target antigen may also enhance binding to similar, non-target molecules.
- Biological System Complexity
Additionally, the complexity of biological systems adds another layer of challenge. Small changes in the conformation or expression of proteins can significantly impact antibody performance. These changes may affect the accessibility or presentation of epitopes, thereby influencing both affinity and specificity. This variability underscores the importance of developing robust methods to assess and optimize antibodies under conditions that closely mimic physiological environments.
Overall, the main challenges in antibody development revolve around managing the trade-offs between affinity and specificity, navigating the vast sequence diversity, and accounting for the dynamic nature of biological systems. Addressing these challenges requires a comprehensive and integrated approach, combining experimental and computational methods to design and select antibodies that meet the stringent requirements of clinical and research applications.
Strategies for Achieving Optimal Balance
Achieving an optimal balance between affinity and specificity in antibody development requires a multifaceted approach. The strategies include site-directed mutagenesis, combinatorial libraries, computational modeling, and iterative selection and affinity maturation, each contributing uniquely to fine-tuning antibody characteristics.
- Site-Directed Mutagenesis
Site-directed mutagenesis is a targeted technique that introduces specific mutations in the antibody’s variable regions, particularly within the complementarity-determining regions (CDRs). This approach allows precise alteration of amino acids that interact directly with the antigen, thus enabling fine-tuning of both affinity and specificity. By modifying key residues, researchers can enhance the binding interactions with the target antigen while minimizing off-target effects. For instance, studies have demonstrated that targeted mutations in the Fc region can significantly alter the binding properties and immune effector functions of antibodies, optimizing their therapeutic potential.
- Combinatorial Libraries
The generation and screening of combinatorial libraries, such as those used in phage, yeast, or ribosome display, offer a robust method for exploring a vast diversity of antibody variants. This approach enables the identification of antibodies with desired properties, such as high affinity and specificity, by selecting from large libraries of variants. The iterative selection process can be further refined by incorporating negative selection steps to eliminate variants that bind non-specifically. This method is especially effective in discovering rare antibody variants with exceptional specificity, as evidenced by the identification of antibodies with improved binding characteristics through advanced display technologies.
- Computational Modeling
Computational modeling and in silico methods have become crucial tools in antibody engineering. These approaches facilitate the prediction of structural and functional outcomes of specific mutations, aiding in the design of antibodies with optimal binding properties. Techniques such as molecular docking simulations provide detailed insights into how antibodies interact with their antigens, helping identify mutations that enhance specificity without compromising affinity. Furthermore, machine learning algorithms can analyze large datasets of antibody sequences and structures, improving the prediction and optimization of binding interactions. Recent advancements have successfully applied these computational methods to enhance the binding affinity and specificity of therapeutic antibodies targeting various antigens.
- Iterative Selection and Affinity Maturation
Affinity maturation involves iterative cycles of mutation and selection aimed at enhancing the binding properties of antibodies. This process typically begins with a diverse library of antibody variants that undergo rounds of selection under increasingly stringent conditions. Variants that retain high-affinity binding are further characterized and optimized. This approach must be carefully managed to prevent the loss of specificity, as the process of increasing affinity can inadvertently lead to cross-reactivity. Advances in structure-based approaches and high-throughput screening technologies have enabled the identification of mutations that enhance affinity while maintaining or even improving specificity.
- Future Directions and Considerations
Balancing affinity and specificity continue to be a challenging aspect of antibody development. The integration of emerging technologies, such as artificial intelligence and next-generation sequencing, promises to further enhance the design and selection of antibodies with tailored properties. As the understanding of antibody-antigen interactions deepens, new methodologies are likely to emerge, refining the ability to achieve the perfect balance of affinity and specificity for various therapeutic and diagnostic applications. These advancements will be critical for developing antibodies that are both highly effective and safe, ensuring their success in clinical and research settings.
Techniques for Enhancing Antibody Affinity and Specificity
Phage Display and Other Library Technologies
Phage display is a pivotal technology in the field of antibody engineering, allowing for the creation and screening of vast libraries of antibody variants. This method involves expressing antibody fragments on the surface of bacteriophages, which can then be screened against target antigens to identify high-affinity binders. The technology’s versatility enables the exploration of a wide range of antibody variants, including those with improved stability and specificity. By using phage display, researchers can fine-tune antibodies to recognize specific epitopes with high precision, making it an essential tool for developing therapeutic antibodies with desired properties.
Additionally, phage display has been complemented by other display technologies, such as yeast and ribosome display. These techniques expand the diversity of antibody libraries and offer alternative platforms for antibody selection, especially useful when phage display libraries encounter limitations. For instance, yeast display allows for the selection of antibodies under conditions closer to physiological environments, which can be advantageous for certain applications.
Computational Approaches and In Silico Modeling
Advancements in computational biology have significantly impacted antibody engineering, particularly in predicting and optimizing antibody-antigen interactions. Computational modeling, including molecular docking and molecular dynamics simulations, has enabled the detailed analysis of how antibodies interact with their targets at the atomic level. These methods allow for the identification of critical residues that contribute to binding affinity and specificity, providing valuable insights for designing mutations.
Molecular docking simulations can predict the binding modes of antibodies to antigens, helping to identify potential sites for mutations that could enhance binding strength. Furthermore, molecular dynamics simulations provide dynamic views of antibody-antigen interactions, revealing how structural flexibility and conformational changes affect binding. These computational tools have been used to achieve significant affinity improvements in therapeutic antibodies, such as the development of variants with enhanced binding to specific antigens.
Machine learning algorithms have further advanced the field by enabling the analysis of large datasets of antibody sequences and structures. These algorithms can predict the effects of mutations on antibody stability and affinity, accelerating the design process. For example, deep learning models have been applied to optimize the specificity of antibodies by predicting cross-reactivity patterns, which is critical for avoiding off-target effects in therapeutic applications.
Site-Directed Mutagenesis and Affinity Maturation
Site-directed mutagenesis is a targeted approach used to introduce specific mutations into the antibody’s variable regions. This technique is instrumental in fine-tuning the interactions between antibodies and antigens by altering key amino acids within the complementarity-determining regions (CDRs). By systematically introducing mutations, researchers can enhance the affinity of antibodies for their targets while minimizing cross-reactivity.
Affinity maturation, often performed through iterative cycles of mutagenesis and selection, further refines antibody properties. This process involves creating libraries of mutated antibodies, which are then subjected to rounds of selection under increasingly stringent conditions. Successful variants are those that exhibit improved binding characteristics, including higher affinity and greater specificity.
Advanced techniques in affinity maturation, such as the use of deep sequencing and high-throughput screening, enable the rapid identification of promising antibody candidates. For example, the incorporation of computational tools into the affinity maturation process allows for the prediction of beneficial mutations, streamlining the optimization of antibody properties.
Overall, these advanced methodologies—spanning phage display, computational modeling, and targeted mutagenesis—constitute a powerful toolkit for engineering antibodies with the desired balance of high affinity and specificity. These approaches continue to evolve, incorporating new technologies and insights that further enhance their effectiveness in developing therapeutic antibodies.
Techniques for Enhancing Antibody Affinity and Specificity
Phage Display and Other Library Technologies
- Overview and Methodology
Phage display is a robust technology that facilitates the generation and screening of vast libraries of antibody fragments (e.g., single-chain variable fragments, scFvs) displayed on the surface of bacteriophages. This technique involves the fusion of the antibody gene to a phage coat protein gene, allowing the expression of the antibody fragment on the phage surface. These phage-displayed libraries can be panned against immobilized antigens to isolate phages binding specifically to the target. After several rounds of selection and amplification, high-affinity binders are identified and further characterized.
Phage display has proven to be highly versatile, capable of generating antibodies against a wide range of antigens, including those that are weakly immunogenic or toxic. This method allows for the rapid identification of antibodies with high affinity and specificity, making it invaluable in both basic research and therapeutic development. Moreover, the use of phage display can be extended to create libraries from human sources, thereby generating fully human antibodies that reduce the risk of immunogenicity in clinical applications.
- Yeast and Ribosome Display
While phage display remains a cornerstone technology, yeast and ribosome display offer alternative methods for antibody selection. Yeast display involves expressing antibodies on the surface of yeast cells, which can be screened using flow cytometry to identify clones with desired binding characteristics. This technique is particularly useful for selecting antibodies under conditions that more closely mimic the human physiological environment, which can be critical for identifying candidates with favorable biophysical properties.
Ribosome display, on the other hand, is a cell-free system that allows the direct selection of proteins based on their binding to a target antigen. This method uses mRNA-ribosome-antibody complexes, which are stable and can be screened for binding activity without the need for cell expression systems. Ribosome display enables the generation of extremely large libraries, thus increasing the chances of identifying rare high-affinity variants.
Computational Approaches and In Silico Modeling
- Molecular Docking
Molecular docking simulations are computational techniques that predict the preferred orientation of an antibody when it binds to an antigen. This approach helps in understanding the binding affinity and specificity of the antibody-antigen complex. By simulating the docking process, researchers can identify key residues involved in the interaction and predict how mutations might enhance or disrupt binding. This predictive capability is critical for designing antibodies with improved properties, especially when experimental determination of structures is challenging.
- Molecular Dynamics Simulations
Molecular dynamics (MD) simulations provide insights into the dynamic behavior of antibody-antigen interactions. Unlike static docking studies, MD simulations consider the flexibility of the molecules and the solvent environment, offering a more realistic view of the binding process. MD simulations can reveal conformational changes upon binding, the stability of the complex, and potential off-target interactions. These insights are invaluable for optimizing both affinity and specificity, as they allow for the exploration of how structural fluctuations impact binding.
- Machine Learning and AI
Machine learning algorithms, including deep learning, have become increasingly important in antibody engineering. These tools can analyze large datasets of antibody sequences and structures to identify patterns associated with high affinity and specificity. By training models on known data, machine learning can predict the effects of mutations and suggest novel sequences with desired properties. For example, AI-driven approaches have been used to optimize antibodies for minimal off-target binding, enhancing specificity without sacrificing affinity.
Site-Directed Mutagenesis and Affinity Maturation
- Site-Directed Mutagenesis
Site-directed mutagenesis is a precise method for introducing specific amino acid changes in the antibody’s variable regions. This technique is used to systematically alter residues in the CDRs to improve binding interactions with the antigen. By targeting residues that directly contact the antigen, researchers can increase the binding strength and specificity of the antibody. For instance, mutations that introduce favorable electrostatic interactions or hydrophobic contacts can significantly enhance affinity.
- Affinity Maturation
Affinity maturation involves iterative cycles of mutagenesis and selection to refine the binding properties of antibodies. The process begins with a diverse library of antibody variants, which undergoes selection for high-affinity binding under stringent conditions. Successful variants are those that not only bind with high affinity but also demonstrate low off-target reactivity. Advanced techniques, such as deep sequencing, are employed to monitor the evolution of antibody libraries, allowing for the rapid identification of promising candidates. Structure-guided approaches and high-throughput screening technologies further enhance the efficiency of affinity maturation, making it possible to achieve substantial improvements in both affinity and specificity.
The combination of these techniques provides a comprehensive toolkit for the development of antibodies with optimized properties. By leveraging these methodologies, researchers can overcome the inherent challenges in antibody engineering, ensuring the production of highly specific and potent therapeutic and diagnostic antibodies. As the field continues to evolve, the integration of emerging technologies will further refine these processes, leading to even more precise and effective antibody-based interventions.
Future Directions in Antibody Development
Emerging Technologies and Techniques
- Next-Generation Sequencing (NGS)
Next-generation sequencing (NGS) has dramatically transformed the landscape of antibody discovery and development. This technology enables the high-throughput sequencing of antibody repertoires, providing a comprehensive view of the diversity present in immune responses. NGS can identify rare, high-affinity antibodies from large libraries, streamlining the discovery process and offering insights into the natural diversity of antibody sequences. This capability is particularly useful for understanding the immune response to pathogens and for discovering therapeutic antibodies with unique properties.
NGS also plays a crucial role in affinity maturation, where it helps track the evolution of antibody variants and identify mutations that improve binding properties without compromising other crucial characteristics such as stability or solubility. The integration of NGS with computational analytics enables the mapping of sequence-function relationships, thereby facilitating the rational design of antibody libraries that are more likely to yield candidates with desirable traits.
- CRISPR/Cas9-Mediated Gene Editing
CRISPR/Cas9 technology has emerged as a powerful tool for precise gene editing, including the modification of antibody genes. In antibody development, CRISPR/Cas9 can be used to introduce specific mutations that enhance antibody properties such as affinity, specificity, and stability. This technique allows for the rapid generation of mutant libraries, which can be screened for improved binding characteristics. Moreover, CRISPR/Cas9 can be utilized to modify the glycosylation patterns of antibodies, potentially enhancing their efficacy and reducing immunogenicity.
The use of CRISPR/Cas9 in combination with NGS and other high-throughput technologies enables a more comprehensive understanding of how specific genetic modifications affect antibody function. This integrated approach can accelerate the development of therapeutic antibodies with optimized properties, tailored to specific clinical needs.
- Trends in Antibody Engineering and Design
The field of antibody engineering is continually evolving, driven by advances in both experimental techniques and computational modeling. A notable trend is the increasing use of artificial intelligence (AI) and machine learning (ML) to predict and optimize antibody sequences. These technologies can analyze vast datasets to identify sequence-structure-function relationships, providing insights that guide the design of antibodies with improved affinity and specificity.
Another significant trend is the development of bispecific antibodies, which can bind to two different antigens simultaneously. This dual-targeting capability enhances the therapeutic potential of antibodies, particularly in oncology, where bispecific antibodies can bring immune cells into close proximity with tumor cells, thereby enhancing immune-mediated tumor cell killing.
The use of smaller antibody fragments, such as single-chain variable fragments (scFvs) and nanobodies, is also gaining traction. These smaller molecules offer advantages in terms of better tissue penetration, reduced immunogenicity, and easier manufacturing processes. Such innovations are crucial for expanding the therapeutic and diagnostic applications of antibodies.
- Collaborative Efforts and Industry-Academic Partnerships
The advancement of antibody technologies increasingly relies on collaborations between academia and industry. These partnerships combine the innovative research capabilities of academic institutions with the large-scale production and commercialization expertise of industry. Such collaborations are essential for translating basic research findings into clinically relevant therapies.
Joint efforts often focus on tackling complex challenges in antibody development, such as improving manufacturability and reducing immunogenicity. For instance, academic labs may develop novel antibody formats or engineering techniques, which are then optimized and scaled up by industry partners for clinical trials and production. These partnerships not only accelerate the development pipeline but also facilitate the sharing of resources and knowledge, fostering innovation in the field.
In summary, the future of antibody development is poised to benefit from cutting-edge technologies and interdisciplinary collaborations. The integration of advanced genetic, computational, and engineering tools will continue to enhance our ability to design and produce antibodies with optimal therapeutic and diagnostic properties. These innovations will be critical for meeting the growing demand for effective, safe, and affordable antibody-based treatments.
ProteoGenix’s Services for Optimal Antibody Development
ProteoGenix is a leading provider of comprehensive antibody development services, leveraging cutting-edge technologies and a deep expertise in antibody engineering. Our offerings are designed to meet the diverse needs of researchers and biotech companies, providing tailored solutions that enhance the affinity and specificity of antibodies. This section highlights our key services, including AI-powered affinity maturation, phage display technology, and comprehensive antibody development, all aimed at delivering high-quality antibodies for various applications.
Overview of ProteoGenix’s Expertise and Capabilities
ProteoGenix specializes in custom antibody production and engineering, employing state-of-the-art technologies to optimize antibody properties. Our team of experts utilizes advanced methodologies, to ensure the development of antibodies with exceptional performance characteristics. We are committed to providing solutions that accelerate research and therapeutic development, from initial antibody discovery to final production.
Affinity Maturation via AI
How AI is Used to Enhance Antibody Affinity at ProteoGenix
At ProteoGenix, we utilize advanced AI technologies to streamline and enhance the antibody affinity maturation process. Our AI-powered service employs machine learning algorithms to analyze large datasets of antibody sequences and structures, rapidly identifying mutations that improve binding affinity and specificity.
- Fast Epitope/Paratope Mapping
Our AI-driven mapping completes within 2 weeks, achieving a 90% reduction in development time compared to traditional methods. This quick turnaround is critical for accelerating research and development.
- AI-Powered Efficiency
The AI analysis efficiently processes data, predicting optimal mutations and significantly reducing the overall development time. This streamlined approach allows us to quickly refine antibody candidates, focusing on those with the best performance characteristics.
- Reliable Validation with XtenCHO™
Using our proprietary XtenCHO™ technology, we validate AI-generated data with robust assays like ELISA and Biacore, ensuring high accuracy without the need for external outsourcing.
- High-Throughput Efficiency
We support large-scale projects with high-throughput antibody production, processing hundreds to thousands of antibodies efficiently in 24-96 well plates.
- Complete IP Ownership
Clients receive full intellectual property rights to their developed antibodies, with guidance from ProteoGenix to verify the uniqueness of their sequences.
- Broad Applicability
Our AI tools are versatile, applicable to a wide range of antibody projects and species, including non-antibody proteins, catering to diverse research needs.
- Proven Success and Expert Supervision
With a high success rate in antibody development, our expert team provides comprehensive support throughout the process, ensuring superior results.
ProteoGenix’s AI-powered affinity maturation service offers a fast, efficient, and reliable way to enhance antibody affinity and specificity, making it an ideal choice for researchers and developers seeking advanced solutions in antibody engineering.
Benefits and Applications of Phage Display at ProteoGenix
How Phage Display Helps Refine Affinity Maturation
At ProteoGenix, we use phage display technology to refine antibody affinity maturation. This method generates extensive libraries of antibody variants, enabling the selection of those with superior binding properties. Through iterative rounds of mutation and selection, phage display identifies and amplifies antibodies with improved affinity and specificity, ensuring the development of highly effective therapeutic antibodies.
Benefits and Applications of Phage Display at ProteoGenix
- Expertise and Experience
28 years of expertise in therapeutic antibody development from leading pharmaceutical companies.
- Large Libraries of Relevant Antibodies
Advanced phage display libraries designed using 3D modeling to limit irrelevant mutations and focus on beneficial mutations, increasing the likelihood of finding highly potent antibodies.
- Advanced Selection Strategies
Incorporation of negative selection techniques to eliminate non-specific binders, ensuring the development of highly specific and effective antibodies.
- IP-Free Solutions
Full intellectual property rights to the affinity-matured antibodies are granted to clients at the end of the project.
- PhD Account Managers
Highly skilled PhD account managers are available to facilitate efficient communication and project management throughout the affinity maturation process.
- One-Stop Solution
Comprehensive services that streamline the process from antibody discovery to clinical application, providing a safe and efficient pathway for therapeutic antibody projects.
At ProteoGenix, we are dedicated to advancing antibody technologies and delivering solutions that meet the highest standards of quality and innovation. Our comprehensive services are designed to support the entire antibody development process, ensuring that our clients receive the best possible products and support.
Explore our range of services and discover how we can assist you in achieving your research and development goals.
Conclusion
The pursuit of achieving the perfect balance between affinity and specificity in antibodies is both a scientific challenge and a technological frontier. The methodologies discussed—ranging from phage display and site-directed mutagenesis to computational modeling and AI-driven affinity maturation—illustrate the diverse and advanced toolkit available for antibody engineering. These technologies not only enhance the precision and efficacy of antibodies but also significantly accelerate the development process, making it possible to quickly bring innovative therapies and diagnostics to market.
ProteoGenix stands at the forefront of this dynamic field, offering comprehensive services that integrate state-of-the-art technologies and decades of expertise. Our approaches in AI-powered affinity maturation and phage display are designed to optimize antibody characteristics, ensuring that the final products meet the highest standards of specificity and affinity. By employing advanced selection strategies and robust validation methods, we provide reliable and highly specific antibodies that cater to a wide range of applications, from therapeutic interventions to diagnostic tools.
The future of antibody development is poised to benefit from emerging technologies such as next-generation sequencing and CRISPR/Cas9-mediated gene editing. These advancements promise to deepen our understanding of antibody-antigen interactions and open new avenues for engineering antibodies with unprecedented specificity and potency. Furthermore, the collaborative efforts between academia and industry will continue to drive innovation, facilitating the translation of scientific discoveries into practical, life-saving applications.
In summary, the continuous evolution of antibody engineering technologies, coupled with ProteoGenix’s expertise, ensures the ongoing enhancement of antibody-based solutions. As we look ahead, the integration of cutting-edge techniques and collaborative frameworks will be crucial in overcoming current limitations and unlocking new potentials in the field of antibody development. This comprehensive approach not only advances scientific knowledge but also has a profound impact on improving human health and disease management.
Resources